Untitled
Topic Introduction
Immersion Freezing of Biological Specimens: Rationale, Principles,and Instrumentation
Guenter P. Resch,1,5 Marlene Brandstetter,1 Angela M. Pickl-Herk,2 Lisa Königsmaier,3Veronika I. Wonesch,1,4 and Edit Urban3
1IMP-IMBA-GMI Electron Microscopy Facility, Institute of Molecular Biotechnology, 1030 Vienna, Austria2Max F. Perutz Laboratories, Medical University of Vienna, 1030 Vienna, Austria3Institute of Molecular Biotechnology, 1030 Vienna, Austria4University of Applied Sciences Wiener Neustadt, 2700 Wiener Neustadt, Austria
Cryo-transmission electron microscopy (cryo-TEM) allows the visualization of biological specimenswithin their native, hydrated environment at nanometer resolution. To prevent the formation of destruc-tive ice crystals, an extremely high cooling rate has to be achieved in the freezing process. For samplesthat are inherently thin enough, ranging from macromolecules to peripheral parts of spread cells, this canbe accomplished by plunging the sample into a cryogen. This approach, known as "immersion freezing,"is an essential preparation technique in structural, molecular, and cell biology. In this article, we discussthe advantages of cryo-EM, the scope of specimens that can be visualized using this technique, themethod of immersion freezing, and the instrumentation available. In particular, we focus on the newsemiautomatic immersion freezer by Leica Microsystems ("EM GP").
RELATED INFORMATION
Technical aspects of immersion freezing are also discussed in Grassucci et al. (2007) and Dobro et al.
(2010). Laboratory protocols are available for Immersion Freezing of Suspended Particles and Cellsfor Cryo-Electron Microscopy (Resch et al. 2011a) and for Immersion Freezing of Cell Monolayersfor Cryo-Electron Tomography (Resch et al. 2011b). Both illustrate the freezing procedure using theexample of the Leica EM GP immersion freezer, with advice for troubleshooting general freezing issuesand the operation of this instrument in particular.
Cold Spring Harbor Protocols
CRYO-ELECTRON MICROSCOPY AND TOMOGRAPHY
In structural biology, imaging of macromolecular complexes embedded in vitrified (amorphous) icebelow recrystallization temperature is the method of choice for visualization in the transmission electronmicroscope (TEM). In contrast to conventional TEM techniques, cryo-electron microscopy obviatespotentially destructive preparative steps such as heavy metal contrasting and removal of water. Thus,it allows one to achieve unprecedented resolution in reconstructions of asymmetric (Chang et al.
2006; Zhang et al. 2008), helical (Egelman 2010; Galkin et al. 2010), icosahedral (Chang et al. 2006),or crystalline (Hite et al. 2010) assemblies after reducing the noise inherent to low-dose images takenof frozen hydrated specimens by averaging corresponding structures.
New developments in cryo-electron tomography (cryo-ET) in the last decade (Baumeister 2002;
Steven and Aebi 2003; McIntosh et al. 2005) now also allow the visualization of pleomorphic structures(Grünewald and Cyrklaff 2006; Norlén et al. 2007) and the crowded environment within cells in threedimensions and at nanometer resolution. Hence, this method has become increasingly important inmolecular and cell biology and is now routinely being used to visualize the thin peripheral parts of adher-ent cells grown on grids (Medalia et al. 2002; Cyrklaff et al. 2007; Urban et al. 2010) and tissues(Al-Amoudi et al. 2007; Bouchet-Marquis et al. 2007). For the first time ever, cryo-ET allows one to
5Corresponding author (
[email protected]).
Cite as: Cold Spring Harb Protoc; 2011; doi:10.1101/pdb.top118
2011 Cold Spring Harbor Laboratory Press
visualize the molecular organization of hydrated cells, potentially without destructive preparativeprocedures.
Immersion Freezing
Physical fixation of the specimen by freezing is the most crucial preparative step in cryo-TEM deservingspecial attention. Foremost, specimens for cryo-EM are frozen without cryo-protectants and, hence,require a cooling rate that prevents the formation of destructive ice crystals. For samples in anaqueous solution with a physiological salt concentration that are thinner than 1 µm (Dubochet andMcDowall 1981; Dubochet 2007), such as suspensions of macromolecules, two-dimensional crystals,or thin parts of cultured cells, this can be achieved by immersion freezing ("plunge freezing"): Thespecimen is applied to a grid, most of the liquid is blotted off with filter paper, and the remainingliquid film containing the sample with a thickness in the magnitude of 100 nm is plunged into acryogen. For this purpose, liquid nitrogen (LN2) is not a suitable coolant, as it is close to its boilingpoint and, hence, forms an insulating gas layer once the sample enters. Instead, liquid ethane,propane, or a mixture of both (Tivol et al. 2008) are commonly used. Beside achieving vitrification, itis crucial to minimize surface contamination on the specimen arising from humidity or impure reagentsand to freeze the biological sample in a physiologically meaningful state.
However, the heat conductivity of water does not allow bigger specimens, such as tissue samples, to
be cooled fast enough to achieve vitrification of the whole specimen at ambient conditions. Hence,approaches delaying the formation of crystalline ice, such as high-pressure freezing (Studer et al.
2001) or self-pressurized rapid freezing (Leunissen and Yi 2009) have to be used to achieve good pres-ervation of structural features.
The simplest version of a device for immersion freezing comprises a forceps holding the grid with the
specimen mounted on a gravity-driven dropping mechanism and an LN2 container surrounding the sec-ondary cryogen (de Carlo 2009). Excess specimen solution is blotted away manually. Many successfulexperiments were and are carried out using these basic devices. However, a number of critical parameterscannot be controlled using these basic techniques. This includes low humidity around the ultrathin filmjust before freezing, which can lead to significant evaporation and, hence, a dramatic increase in the con-centration of solutes (Cyrklaff et al. 1990; Frederik and Hubert 2005), limiting the significance and repro-ducibility of the results obtained. Likewise, the temperature of this ultrathin liquid layer can be influencedeasily by the environment, particularly the cold gaseous nitrogen (GN2) from the container just below.
A defined temperature of the biological specimen before freezing is, however, essential, both in singleparticle analysis to decrease thermal fluctuation or to make certain intermediate states accessible(Fischer et al. 2010), as well as in cell monolayers to keep physiological processes active. Furthermore,on basic plunge freezers the secondary cryogen needs to be warmed up frequently for melting, asboth ethane and propane alone freeze at LN2 temperature (Tivol et al. 2008).
Cold Spring Harbor Protocols
To more tightly control the freezing conditions, these devices were soon fitted with environmental
control (Cyrklaff et al. 1990; White et al. 2003; Arnal et al. 2009), heaters to keep the secondary cryogen
liquid, or automatic blotting mechanisms (Cyrklaff et al. 1990; White et al. 2003). Others were adapted
for time-resolved experiments (White et al. 2003) or operated in the cold room to reduce evaporation
(Grassucci et al. 2007). However, success and reproducibility of the experiments highly depend onthe skills of the individual performing them, in particular on the instruments that require manual blotting.
Semiautomatic Immersion Freezers
In the last decade, the first commercial semiautomatic plunge freezers were introduced, aiming to makeimmersion freezing a more straightforward and reproducible process. One prominent example pro-duced by FEI (Eindhoven, Netherlands) is the Vitrobot, currently available in the Mk IV version. Thisinstrument (Frederik and Hubert 2005) features an environmental chamber with controlled temperatureand humidification that surrounds the specimen before freezing and a double-sided automatic blottingmechanism using two pieces of filter paper. The intensity of the blot can be controlled by the position ofthe grid within the wedge of filter papers. The Vitrobot also uses a cryogen container that prevents freez-ing of the secondary cryogen by insulating it from LN2. It allows an automated transfer of the frozen gridfrom the secondary cryogen to the LN2 dewar, where the box for grid storage is located. The instrumentis controlled via a touchscreen, and a number of parameters can be adjusted. Laboratory protocols thatdescribe immersion freezing with this instrument are available (Iancu et al. 2007; Frederik et al. 2009).
Cold Spring Harbor Protocols
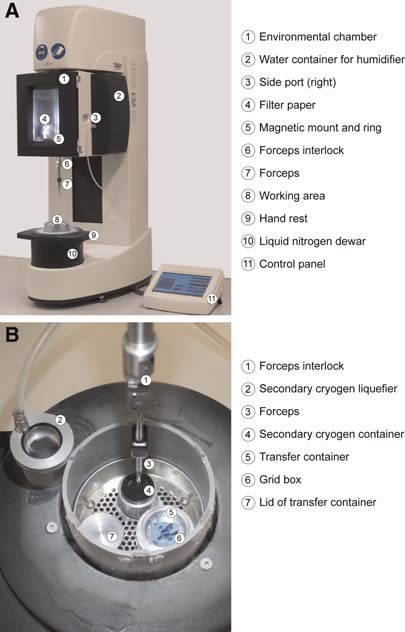
Another instrument, the Cp3, is being produced by Gatan (http://www.gatan.com/). This device's
environmental chamber allows humidification via a sponge and monitoring of humidity and tempera-ture via a sensor. The blotting mechanism is pneumatic and can be used either single- or double-sided.
A temperature control of the secondary cryogen avoids freezing.
In 2009, Leica Microsystems (http://www.leica-microsystems.com) launched a new immersion
freezer developed in close collaboration with our lab, the Leica EM GP ("grid plunger") (Fig. 1). BothImmersion Freezing of Suspended Particles and Cells for Cryo-Electron Microscopy (Resch et al.
2011a) and Immersion Freezing of Cell Monolayers for Cryo-Electron Tomography (Resch et al.
2011b) are tailored for the EM GP; its key features and the steps involved in a freezing cycle are brieflyintroduced here. In addition to standard components like an environmental chamber (+4 to +60˚C,
up to 99% relative humidity) that lowers onto the LN2 dewar before plunging, an easy-to-use computerinterface operated via a touch screen (Fig. 2), and a controlled secondary cryogen heating, the GP incor-porates a number of innovations.
A notable difference compared with the other semiautomatic immersion freezers available is that
the GP primarily uses single-sided blotting. As this is the way most microscopists blot manually, experi-ence obtained from manual blotting can be easily transferred to the GP. Furthermore, unilateral blottingfrom the "back" side of the grid is the preferred way to prepare frozen hydrated cell monolayers. If speci-mens require blotting from both sides, the grids can be rotated 180˚ to be blotted from both sides
consecutively. The instrument uses a blotting sensor to measure the point of contact between specimenand filter paper (Lihl and Resch 2010) to exert comparable pressure on each grid to be blotted. Even withbent grids or filter paper that is strongly bent due to high humidity, very reproducible results canbe obtained.
The GP implements a number of measures to prevent contamination of the specimen by atmos-
pheric humidity. This includes balanced pressures between the environmental chamber and theworking area, as well as a constant flow of GN2 to keep humidity out of the critical working area. Theelaborate anticontamination system makes it possible to freeze many grids in a row (at least 20)without accumulating too much ice, even without refilling the LN2 in the main dewar. It might be a
Cold Spring Harbor Protocols
FIGURE 1. (A) Overview of the main unit without the (optional)viewing system mounted. The environmental chamber is inthe lifted position to load the quick-lock forceps. For freezing,it is lowered directly onto the working area. (B) Close-up viewof the working area with a forceps in freezing position. Notethe lack of contamination inside the working area, while theoutside is already frosted with ice after some time.
Cold Spring Harbor Protocols
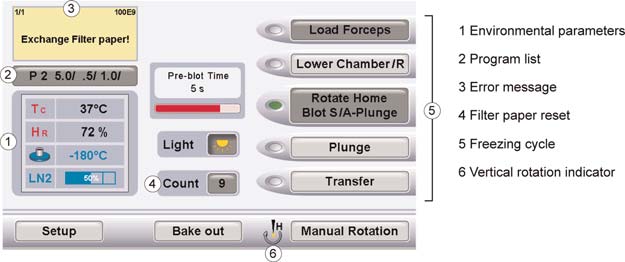
FIGURE 2. Screen shot of the control panel.
disadvantage in a very busy lab, however, that the LN2 dewar and the working area cannot be swappedfor a warm and frost-free setup as on other instruments. On the GP, a full bake-out, completed within atmost 1.5 h, is required after some hours of work to remove ice contamination.
The following summary covers the individual stages of freezing one grid according to the user inter-
face (Fig. 2), the input required from the user, and the sequence of actions by the instrument. Settingstypical for biological specimens (i.e., single blot and automatic plunging of the specimen) are assumed.
The GP Freezing Cycle
1. "Load Forceps" (Fig. 1)
Instrument: Forceps holder lifts up to receive the forceps.
Instrument: Humidifier switches on.
User: Inserts quick lock forceps with the bare grid or the grid carrying cell monolayer.
2. "Lower Chamber"
Instrument: Environmental chamber lowers onto the LN2 dewar/working area.
(Instrument, optional: Forceps rotates around the vertical axis.)Instrument: Shutter from environmental chamber to working area closes.
(User, optional: Applies specimen onto the grid via one of the side ports.)
3. "(Rotate Home)/Blot/A-Plunge"
(Instrument, optional: Forceps rotates around the vertical axis.)
Cold Spring Harbor Protocols
(Instrument, optional: Pre-blot time elapses.)
Instrument: Humidifier switches off.
Instrument: Blotting of the grid.
(Instrument, optional: Post-blot time elapses.)
4. (Inactive with A-Plunge setting: "Plunge")
Instrument: Shutter from environmental chamber to working area opens.
Instrument: Automatic plunging of the grid into the secondary cryogen.
Instrument: Environmental chamber lifts up, grid remains in secondary cryogen.
5. (Optional: "Transfer")
(Instrument, optional: The grid is lifted to the transfer position.)
The GP was successfully used for freezing a wide range of biological specimens such as viruses, liposomes(Hasanovic et al. 2011), Salmonella type III secretion system needle complex (Wagner et al. 2010), andmonolayers of different cell lines (Urban et al. 2010). The methodology used in these studies is detailed inImmersion Freezing of Suspended Particles and Cells for Cryo-Electron Microscopy (Resch et al.
2011a) and Immersion Freezing of Cell Monolayers for Cryo-Electron Tomography (Resch et al.
2011b). Although the instrument can also be used to freeze industrial specimens such as emulsions orpolymers for cryo-EM, these samples are outside the scope of the authors' research and will not bediscussed.
Cold Spring Harbor Protocols
We thank Hannes Tkadletz for his support with graphics and Ian Lamswood, Dr. Reinhard Lihl, AndreasNowak, and Dr. Ruwin Pandithage from Leica Microsystems for discussions. The feedback of Dr. Vic Smallregarding this manuscript is appreciated. The work of G.P.R. and M.B. was supported by the Cityof Vienna/Zentrum fuer Innovation und Technologie through the Spot of Excellence grant "Center ofMolecular and Cellular Nanostructure." L.K. gratefully acknowledges scholarship support from theBoehringer Ingelheim Fonds; E.U. is supported by the Austrian Science Fund (FWF project P21292-B09 to J.V. Small).
Al-Amoudi A, Diez DC, Betts MJ, Frangakis AS. 2007. The molecular
Hasanovic A, Winkler R, Resch GP, Valenta C. 2011. Modification of the
architecture of cadherins in native epidermal desmosomes.
conformational skin structure by treatment with liposomal formu-
Nature 450: 832–837.
lations and its correlation to the penetration depth of aciclovir.
Arnal I, Malleter M, Chretien D. 2009. Vitrification of dynamic micro-
Eur J Pharm Biopharm. doi: 10.1016/ejbp.2011.01.018.
tubules. In Handbook of cryo-preparation methods for electron
Hite RK, Schenk AD, Li Z, Cheng Y, Walz T. 2010. Collecting electron
microscopy (ed. Cavalier A et al.), CRC Press, Boca Raton, FL.
crystallographic data of two-dimensional protein crystals. Methods
Baumeister W. 2002. Electron tomography: towards visualizing the
Enzymol 481: 251–282.
molecular organization of the cytoplasm. Curr Opin Struct Biol
Iancu CV, Tivol WF, Schooler JB, Dias DP, Henderson GP, Murphy GE,
12: 679–684.
Wright ER, Li Z, Yu Z, Briegel A, et al. 2007. Electron cryotomo-
Bouchet-Marquis C, Zuber B, Glynn AM, Eltsov M, Grabenbauer M,
graphy sample preparation using the Vitrobot. Nat Protoc 1:
Goldie KN, Thomas D, Frangakis AS, Dubochet J, Chretien D.
2007. Visualization of cell microtubules in their native state. Biol
Leunissen JLM, Yi H. 2009. Self-pressurized rapid freezing (SPRF): a
Cell 99: 45–53.
novel cryofixation method for specimen preparation in electron
Chang J, Weigele P, King J, Chiu W, Jiang W. 2006. Cryo-EM asym-
microscopy. J Microsc 235: 25–35.
metric reconstruction of bacteriophage P22 reveals organization
Lihl R, Resch G. 2010. Vorrichtung und Verfahren zum Präparieren von
of its DNA packaging and infecting machinery. Structure 14:
Proben. Patent AT00000000507079B1.
McIntosh R, Nicastro D, Mastronarde D. 2005. New views of cells in
Cyrklaff M, Adrian M, Dubochet J. 1990. Evaporation during prep-
3D: an introduction to electron tomography. Trends Cell Biol 15:
aration of unsupported thin vitrified aqueous layers for cryo-
electron microscopy. J Electron Microsc Tech 16: 351–355.
Medalia O, Weber I, Frangakis AS, Nicastro D, Gerisch G, Baumeister W.
Cyrklaff M, Linaroudis A, Boicu M, Chlanda P, Baumeister W, Griffiths
2002. Macromolecular architecture in eukaryotic cells visualized by
G, Krijnse-Locker J. 2007. Whole cell cryo-electron tomography
cryoelectron tomography. Science 298: 1209–1213.
reveals distinct disassembly intermediates of vaccinia virus. PLoS
Norlén L, Masich S, Goldie KN, Hoenger A. 2007. Structural analysis of
One 2: e420. doi: 10.1371/journal.pone.0000420.
vimentin and keratin intermediate filaments by cryo-electron
de Carlo S. 2009. Plunge Freezing (Holey Carbon Method). In
tomography. Exp Cell Res 313: 2217–2227.
Handbook of cryo-preparation methods for electron microscopy
Resch GP, Brandstetter M, Königsmaier L, Urban E, Pickl-Herk AM.
(ed. Cavalier A et al.), CRC Press, Boca Raton, FL.
2011a. Immersion freezing of suspended particles and cells for
Dobro MJ, Melanson LA, Jensen GJ, McDowall AW. 2010. Plunge freez-
cryo electron microscopy. Cold Spring Harb Protoc doi: 10.1101/
ing for electron cryomicroscopy. Methods Enzymol 481: 63–82.
Dubochet J. 2007. The physics of rapid cooling and its implications for
Resch GP, Brandstetter M, Wonesch VI, Urban E. 2011b. Immersion
Cold Spring Harbor Protocols
cryoimmobilization of cells. Methods Cell Biol 79: 7–21.
freezing of cell monolayers for cryo electron tomography. Cold
Dubochet J, McDowall AW. 1981. Vitri
fication of pure water for elec-
Spring Harb Protoc doi: 10.1101/pdb.prot5643.
tron microscopy. J Microsc 124: RP3
Steven AC, Aebi U. 2003. The next ice age: cryo-electron tomography
Egelman EH. 2010. Reconstruction of helical filaments and tubes.
of intact cells. Trends Cell Biol 13: 107–110.
Methods Enzymol 482: 167–183.
Studer D, Graber W, Al-Amoudi A, Eggli P. 2001. A new approach for
Fischer N, Konevega AL, Wintermeyer W, Rodnina MV, Stark H. 2010.
cryofixation by high-pressure freezing. J Microsc 203: 285–294.
Ribosome dynamics and tRNA movement by time-resolved elec-
Tivol WF, Briegel A, Jensen GJ. 2008. An improved cryogen for plunge
tron cryomicroscopy. Nature 466: 329–333.
freezing. Microsc Microanal 14: 375–379.
Frederik PM, Hubert DH. 2005. Cryoelectron microscopy of liposomes.
Urban E, Jacob S, Nemethova M, Resch GP, Small JV. 2010. Electron
Methods Enzymol 391: 431–448.
tomography reveals unbranched networks of actin filaments in
Frederik PM, de Haas F, Storms MMH. 2009. Controlled Vitrification.
lamellipodia. Nat Cell Biol 12: 429–435.
In Handbook of cryo-preparation methods for electron microscopy
Wagner S, Königsmaier L, Lara-Tejero M, Lefebre M, Marlovits TC,
(ed. Cavalier A et al.), CRC Press, Boca Raton, FL.
Galán JE. 2010. Organization and coordinated assembly of
Galkin VE, Schmied WH, Schraidt O, Marlovits TC, Egelman EH. 2010.
the type III secretion export apparatus. Proc Natl Acad Sci 107:
The structure of the Salmonella typhimurium type III secretion
system needle shows divergence from the flagellar system. J Mol
White HD, Thirumurugan K, Walker ML, Trinick J. 2003. A second gen-
Biol 396: 1392–1397.
eration apparatus for time-resolved electron cryo-microscopy using
Grassucci RA, Taylor DJ, Frank J. 2007. Preparation of macromolecular
stepper motors and electrospray. J Struct Biol 144: 246–252.
complexes for cryo-electron microscopy. Nat Protoc 2: 3239–3246.
Zhang X, Settembre E, Xu C, Dormitzer PR, Bellamy R, Harrison SC,
Grünewald K, Cyrklaff M. 2006. Structure of complex viruses and
Grigorieff N. 2008. Near-atomic resolution using electron cryo-
virus-infected cells by electron cryo tomography. Curr Opin Micro-
microscopy and single-particle reconstruction. Proc Natl Acad Sci
biol 9: 437–442.
105: 1867–1872.
Cold Spring Harbor Protocols
Source: http://cbi.ibp.ac.cn/workshop2015_AWCCEM/material/Resch11c-Topic%20Introduction-Immersion%20Freezing%20of%20Biological%20Specimens%20Rationale%20Principles%20and%20Instrumentation.pdf
This page intentionally left blank Laurie Rozakis, Ph.D. The State University of New York Farmingdale State College New York Chicago San Francisco Lisbon London Madrid Mexico City Milan New Delhi San Juan Seoul Singapore Sydney Toronto Copyright © 2007, 1999 by The McGraw-Hill Companies, Inc. All rights reserved. Manufacturedin the United States of America. Except as permitted under the United States Copyright Act of1976, no part of this publication may be reproduced or distributed in any form or by any means,or stored in a database or retrieval system, without the prior written permission of the publisher.
Healthcare European Pharmaceuticals Novartis It's an uphill climb to the bottom from Equal Weight Earnings and execution headaches: With the sector at a 30% premium to the EU market (1yr fwd PE) earnings and execution uncertainties are not en vogue, which is why we now rate Novartis a relative UW (was EW). The company's top-line growth is >50% dependent on two launch products (Entresto in heart failure and Cosentyx in