Rapid detection of genetically modified organisms on a continuous-flow polymerase chain reaction microfluidics
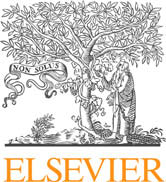
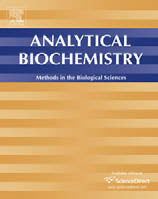
Analytical Biochemistry 385 (2009) 42–49
Contents lists available at
Analytical Biochemistry
Rapid detection of genetically modified organisms on a continuous-flowpolymerase chain reaction microfluidics
Yuyuan Li, Da Xing *, Chunsun Zhang
MOE Key Laboratory of Laser Life Science and Institute of Laser Life Science, South China Normal University, No. 55, Zhongshan Avenue West, Tianhe District,Guangzhou 510631, People's Republic of China
The ability to perform DNA amplification on a microfluidic device is very appealing. In this study, a com-
Received 29 July 2008
pact continuous-flow polymerase chain reaction (PCR) microfluidics was developed for rapid analysis of
Available online 26 October 2008
genetically modified organisms (GMOs) in genetically modified soybeans. The device consists of threepieces of copper and a transparent polytetrafluoroethylene capillary tube embedded in the spiral channel
fabricated on the copper. On this device, the P35S and Tnos sequences were successfully amplified within
Polymerase chain reaction
9 min, and the limit of detection of the DNA sample was estimated to be 0.005 ng ll1. Furthermore, a
duplex continuous-flow PCR was also reported for the detection of the P35S and Tnos sequences in GMOs
simultaneously. This method was coupled with the intercalating dye SYBR Green I and the melting curve
Genetically modified organismsSYBR Green I
analysis of the amplified products. Using this method, temperature differences were identified by the
Melting curve analysis
specific melting temperature values of two sequences, and the limit of detection of the DNA samplewas assessed to be 0.01 ng ll1. Therefore, our results demonstrated that the continuous-flow PCR assaycould discriminate the GMOs in a cost-saving and less time-consuming way.
Ó 2008 Elsevier Inc. All rights reserved.
Since the beginning of recombinant DNA technology, geneti-
cessed and heat-treated food products. Therefore, polymerase
cally modified organisms (GMOs)have brought many advantages
chain reaction (PCR) based on detection of DNA is the most
such as considerable improvement in the yield and quality of crops
widespread method . For example, the matrix approach pro-
and enhancement of the nutritional quality of plants With the
posed by INRA (French National Institute for Agriculture Research)
widespread use of GMOs in food production, labeling regulations
in 1999 for the GMOchips program was a combination of PCR and
have been established in some countries to protect the rights of con-
hybridization to detect authorized and unauthorized GMOs .
sumers, producers, and retailers . For example, the European Un-
Currently, a large number of GMOs share the same promoter of
ion has been regulating the labeling of genetically modified (GM)
the subunit 35S of ribosomal RNA of cauliflower mosaic virus
foods since 1997 (regulation 258/97/EC).
(P35S) and the nopaline synthetase terminator (Tnos) from Agro-
To verify compliance with labeling requirements, several sys-
bacterium tumefaciens . Thus, in practice, they are widely
tems for the detection of GMOs have already been developed and
amplified to detect whether the tissues contain GM components.
described . Up to now, the detection molecules of GMOs have in-
Today most PCR amplifications are carried out on a conven-
cluded DNAs, RNAs, and proteins . DNA is a relatively stable
tional PCR machine using a heating/cooling block of large heat
molecule, allowing its extraction from all kinds of tissues due to
capacity that often has a number of technical frailties and ulti-
uniqueness of DNA in every type of cell and its analysis from pro-
mately restricts the speed and efficiency of the amplification pro-cess For rapid PCR, the low heat capacity of the entirePCR system is important, and performing the rapid PCR is difficult
* Corresponding author. Fax: +86 20 85216052.
in a conventional PCR instrument using a heating/cooling block of
E-mail address: (D. Xing).
large capacity. Therefore, some research groups have made an at-
1 Abbreviations used: GMO, genetically modified organism; GM, genetically mod-
tempt to develop microfluidics-based PCR biomicrofluidic devices
ified; PCR, polymerase chain reaction; P35S, 35S promoter; Tnos, the nopaline
Currently, there are two formats of microfluidic PCR de-
synthetase terminator; l-TAS, micro total analytical system; MCA, melting curveanalysis; PID, proportional/integral/derivative; PTFE, polytetrafluoroethylene; dNTP,
vices : microchamber stationary PCR and continuous-flow
deoxynucleotide triphosphate; ddH2O, doubly deionized H2O; BSA, bovine serum
PCR. The former is the miniaturization of conventional PCR in nat-
albumin; BPB, bromophenol blue; EDTA, ethylenediaminetetraacetic acid; CV,
ure where the PCR mixture is stationary in the chamber and the
coefficient of variation; SD, standard deviation; SVR, surface-to-volume ratio; LOD,
temperature is cycled repeatedly . However, the chamber
limit of detection; ELISA, enzyme-linked immunosorbent assay; RT–PCR, real-time
stationary PCR microfluidics lacks the flexibility to change the
0003-2697/$ - see front matter Ó 2008 Elsevier Inc. All rights reserved.
doi:10.1016/j.ab.2008.10.028
Rapid detection of genetically modified organisms / Y. Li et al. / Anal. Biochem. 385 (2009) 42–49
reaction rate, resulting in more cycling and heating time. More-
gion is twice the size of the other two zones. The three temperature
over, to reduce the reaction time and power consumption, the sys-
zones are separated from each other by thermally insulating sheets
tem thermal mass must be optimized considerably . Compared
that have a thickness of 3 mm. Each zone includes one larger cen-
with microchamber stationary PCR, the continuous-flow PCR has a
tral hole (8 mm diameter) for the resistance cartridge heater (8
few advantages . The heating and cooling rates for PCR ampli-
mm diameter, 100 mm length, 300 W, Guangzhou Haoyi Thermal
fication are confined not by the system thermal mass but rather by
Electronics Factory, Guangzhou, China) and two small holes (1
the flow velocities of PCR mixture in a microchannel, the PCR sam-
mm diameter and 10 mm depth) for the K-type thermocouples
ple solution does not suffer from large evaporation at high temper-
(0.005 inch diameter, Omega Engineering, Stamford, CT, USA).
atures, it is easier to integrate other analytical elements to develop
The thermocouples were connected to a data acquisition system
the micro total analytical system (l-TAS) and this format
(model PCI 4351, National Instruments, Austin, TX, USA) that con-
can realize high-throughput PCR amplification by continuously
verted the analog signal to a digital one. To control the tempera-
providing various biological sample plugs that could save much
tures for denaturation at 94 °C, annealing at 56 °C and extension
time and labor. Currently, the structural styles of the continuous-
at 72 °C, a computer received the temperature signal through a
flow PCR microfluidics can be divided into three main categories:
PCI-4351 interface (National Instruments) and determined the
(i) the serpentine channel continuous-flow PCR , a con-
power input to the heater using a homemade fuzzy proportional/
tinuous-flow format that is based on the work of Nakano and
integral/derivative (PID) control algorithm that was programmed
coworkers and Kopp and coworkers ; (ii) the spiral chan-
with LabVIEW 8.0 (National Instruments).
nel continuous-flow PCR which consists of a ‘‘circular"
A 5.2-m long transparent polytetrafluoroethylene (PTFE) capil-
arrangement of the three zones to generate the sequence of dena-
lary (0.5 mm i.d./0.9 mm o.d., Wuxi Xiangjian Tetrafluoroethylene
turation, annealing, and elongation; and (iii) the straight channel
Product, Wuxi, China) includes the inlet for samples injection and
oscillatory-flow PCR a type of flow-through PCR microflui-
the outlet for product collection. It enters the cylinder through a
dics that consists of a capillary tube, heater zones, an optical win-
hole (1.5 mm diameter) crossing the annealing and denaturation
dow, and so on.
zones, providing an initial denaturation step. Then the capillary
In complying with ISO/DIS 24276, successive simplex DNA
is wound 35 cycles in the spiral channel (1.1 mm width and 1.1
amplification and duplex PCR were used in the study for GMO
mm depth). The capillary exits the cylinder through a hole (1.5
detection to save considerable time and effort. For the purpose of
mm diameter) in the elongation region, providing an additional
identifying the 195- and 180-bp sequences simultaneously, melt-
extension on the 35th cycle. The inlet and outlet lengths of the cap-
ing curve analysis (MCA) was exploited in duplex PCR, which
illary both were approximately 0.35 m. When the PCR cycles were
was based on SYBR Green I, an intercalating dye that is widely used
increased to 45, the length of PTFE capillary was changed to 6.5 m.
. With a high affinity for double-stranded DNA and enhanced
This device can perform up to 60 cycles.
fluorescence on DNA binding , SYBR Green I offers a good alter-native as continuous monitoring of the fluorescence of amplicons
Reagents and samples
along with gradient changes in temperature. That can be used todetermine the melting curves of these sequences. Although fluo-
PCR reagents, 10 Taq DNA polymerase buffer (500 mM KCl
rescence in the SYBR Green I reaction is not sequence specific, it
and 100 mM Tris–HCl, pH 8.8), MgCl2 solution (25 mM), and ther-
is possible to identify the amplified products by their melting tem-
mostable Taq polymerase (5 U ll1) were purchased from Bio Basic
perature (Tm). Because the melting curve of a product is dependent
(BBI, Ontario, Canada). Deoxynucleotide triphosphates (dNTPs,
on GC content, length, and sequence, PCR products can be distin-
10 mM each of dATP, dGTP, dCTP, and dTTP) and PCR primers
guished by MCA .
() were obtained from Shanghai Sangon BiologicalEngineering & Technology Services (SSBE, Shanghai, China). The
Materials and methods
doubly deionized H2O (ddH2O) was provided by Tiangen Biotech(Beijing, China). GM soybeans were gifts from Guangdong Entry–
Exit Inspection and Quarantine Bureau (Guangzhou, China), andthe genomic DNA of soybean was extracted by using a plant geno-
The continuous-flow PCR microfluidics device, depicted sche-
mic DNA extraction kit (Win Honor Bioscience [South], Guangzhou,
matically in , was manufactured by Automation Engineering
R&M Centre (AERMC, Guangdong Academy of Sciences, Guangz-
Bovine serum albumin (BSA, fraction V, purity P 98%, biotech-
hou, China). It consists of a 4-cm diameter and 10-cm length cop-
nology grade, cat. no. 735094), which was used to dynamically coat
per cylinder machined into three pieces corresponding to the
the inner surface to decrease the surface adsorption , was pur-
denaturation, annealing, and extension regions. The extension re-
chased from Roche Diagnostics (Mannheim, Germany). Sodiumhypochlorite solution, which was used to remove the residualDNA from the microchannel after each of the amplifications, wasobtained from Guanghua Chemical Factory (Guangzhou, China).
GoldView dye and SYBR Green I were purchased from SBS Gene-tech (Beijing, China). The DNA markers, which contain 2000-,1000-, 750-, 500-, 250-, and 100-bp DNA fragments, were obtainedfrom Win Honor Bioscience (South).
Continuous-flow PCR amplification
For the continuous-flow PCR amplification, 25 ll of PCR mixture
consists of 1 PCR buffer, 1.5 mM MgCl2, 0.2 mM of each dNTP, theprimer pair (0.5 lM each), 5 ng ll1 soybean genomic DNA, 0.05 Ull1 Taq DNA polymerase, and 0.025% (m/V) BSA. The PCR mixturewas introduced into the capillary from the inlet and compelled by
Fig. 1. Schematic diagram of continuous-flow PCR microfluidics.
the precision syringe pump (cat. no. CZ-74901-15, Cole–Parmer
Rapid detection of genetically modified organisms / Y. Li et al. / Anal. Biochem. 385 (2009) 42–49
Table 1Primers used in the study
Amplicon size (bp)
Instrument, Vernon Hills, IL, USA) to flow continuously through the
tively remove the residual DNA from the microchannel, and each
microchannel. The 0.2-ml thin-walled polypropylene tube was
PCR product could also be collected separately for further analysis.
used to collect the products and stored at 4 °C for further analysis.
The capillary was to be used for a number of amplification
Duplex PCR on the continuous-flow device
experiments, so a cleaning method was developed to validly re-move the residual PCR mixtures from the microchannel. The meth-
The PCR mixture (25 ll) consisted of 1 PCR buffer, 1.5 mM
od consists of a 100-ll purification wash of 5% sodium
MgCl2, 0.2 mM of each dNTP, 5.0 ng ll1 soybean genomic DNA,
hypochlorite solution and a 100-ll cleaning of deionized water,
two pairs of primers (the final concentration of 200 nM for the
followed by a 50-ll rinse of 1 PCR buffer.
P35S sequence and 400 nM for the Tnos sequence), 0.1 U ll1
To compare amplification characteristics (speed, specificity, and
Taq DNA polymerase, and 0.05% (m/V) BSA. The flow velocity
yield), the positive control PCR was performed on a conventional
was controlled at 5 mm s1 by the syringe. To study the effect of
PCR apparatus, which was a commercial Mastercycler gradient
the amount of initial DNA sample on duplex PCR, amplifications
PCR machine (Eppendorf, Hamburg, Germany). The cycling proce-
were also performed using a wide range of DNA concentrations
dures were set as follows: an initial step of denaturation at 94 °C
from 0.005 to 5.0 ng ll1.
for 3 min; 35 cycles of denaturation at 94 °C for 30 s, anneal at55 °C for 30 s, and elongation at 72 °C for 1 min; and final exten-
Analysis of amplification products
sion at 72 °C for 3 min. In total, the above program requires85 min. To validate the amplification, negative controls (without
Here 8 ll of each PCR product with 1.6 ll of 6 loading buffer
template DNA) were also performed to ascertain whether the
was separated by agarose gel electrophoresis. Loading buffer con-
residual contamination existed. All experiments in this work were
tained 30 mM ethylenediaminetetraacetic acid (EDTA), 36% (v/v)
repeated three times to verify the accuracy of the experiments.
glycerol, 0.05% (w/v) xylene cyanol FF, and 0.05% (w/v) BPB. Thegel was prepared with 1.5% agarose in 0.5 TBE buffer containing
Continuous-flow PCR at various flow rates
0.5 ll ml1 GoldView as fluorescence dye. The running conditionswere constant voltage at 100 V. After electrophoresis, which took
To verify the rapid detection capability of the microfluidic de-
approximately 30 min, the relative amounts of PCR products were
vice, PCR of the 195-bp sequence in the P35S and the 180-bp se-
analyzed by image analysis software (Quantity One, Bio-Rad, Her-
quence in the Tnos of GM soybeans was performed at different
cules, CA, USA). The DL 2000 DNA markers, which contain 2000-,
flow rates of the corresponding PCR mixture through the thermal
1000-, 750-, 500-, 250-, and 100-bp DNA fragments, were used
cycling capillary. The flow velocity was controlled by the syringe,
as standards for the evaluation of the gels.
ranging from 2.0 to 15.0 mm s1. The components in the PCR mix-
After continuous-flow PCR, MCA using SYBR Green I was per-
ture are the same as those mentioned above.
formed with the LightCycler (Roche Diagnostics, Mannheim, Ger-many). The products with SYBR Green I (1) were heated to
Continuous-flow PCR at various initial DNA concentrations
95 °C during 15 s, cooled at 60 °C for 20 s, and then slowly heatedback to 95 °C at a rate of 0.1 °C s1. Obtained fluorescence signals
The continuous-flow PCR was performed using a wide range of
were monitored continuously during the slow warming-up gradi-
DNA concentrations from 0.001 to 7.5 ng ll1. The 180-bp se-
ent and slowed to a decreasing curve with a sharp fluorescence
quence was amplified at a flow rate of 3.5 mm s1, then analyzed
drop near the denaturation temperature. Plotting the negative der-
by the gel agarose electrophoresis, and amplified at a flow rate of
ivate of the fluorescence over the temperature versus the temper-
5.0 mm s1, then analyzed by melting curves. These experiments
ature (–dF/dT vs. T) generated peaks from which the Tm values of
were also performed on the conventional PCR machine, which
the products were calculated
was a commercial Mastercycler gradient PCR machine, and the cy-cling procedures were the same as the descriptions mentionedabove.
Results and discussion
Segmented-flow PCR of different DNA samples
Effect of various flow rates on the continuous-flow PCR amplification
PCR mixtures (25 ll) were prepared with different DNA samples
The cycling rate of the continuous-flow PCR thermocycler de-
and PCR primers for amplifying the 180-bp sequence and/or 195-
pends, to some extent, on the flow rate of the PCR mixture, the sub-
bp sequence. Different DNA samples in PCR mixtures included
strate material, and the size of the microchannel . Therefore, an
the genomic DNA of GM and non-GM soybeans. Then these PCR
obvious characteristic of the continuous-flow PCR amplification is
mixtures were sequentially injected into the capillary, and the neg-
that thermocycling rates of PCR amplification can be regulated
ative control was injected after the PCR mixture containing the
by changing the flow rates of the PCR mixture through the flow
genomic DNA of non-GM soybeans, which was amplified to ascer-
channel. The upper panel of A shows the gel electrophoresis
tain whether the resulting amplicon was a product of residual con-
results of the 195-bp sequence in P35S obtained at flow rates rang-
tamination. Then these PCR mixtures were driven to flow through
ing from 2.0 to 15.0 mm s1, whereas the lower panel of A
the thermal cycling capillary at a flow velocity of 3.5 mm s1. Be-
shows the relative amounts of obtained PCR products as deter-
tween each sample introduced, we interposed small air gaps, 15 ll
mined by image analysis software. Values were normalized to
of 1 PCR buffer containing 0.4 bromophenol blue (BPB) buffer,
the fluorescence of the positive PCR products from the conven-
and then small air gaps. This cleaning method was used to effec-
tional PCR machine (100%, lane 1). With the increase of flow veloc-
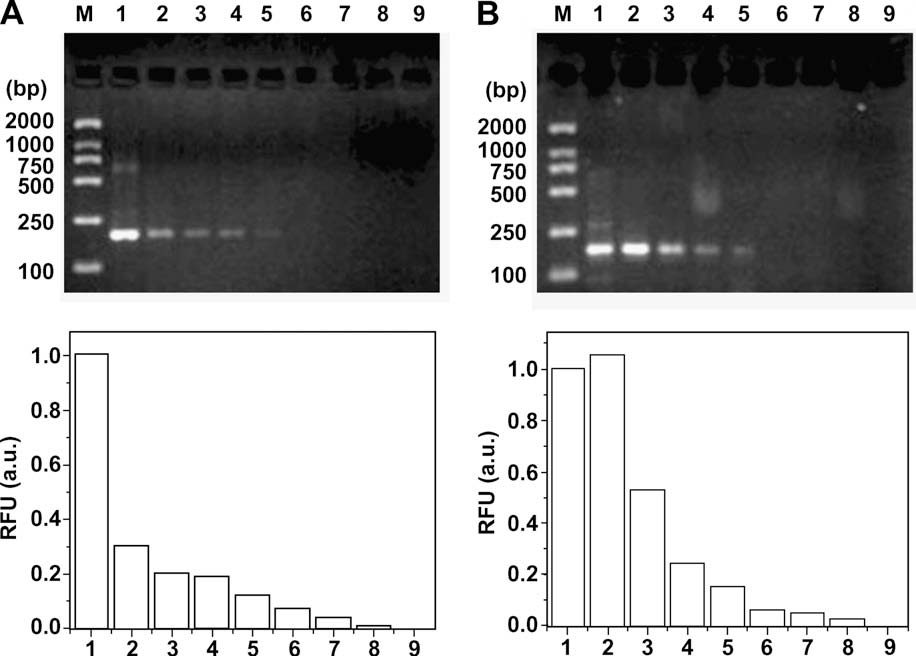
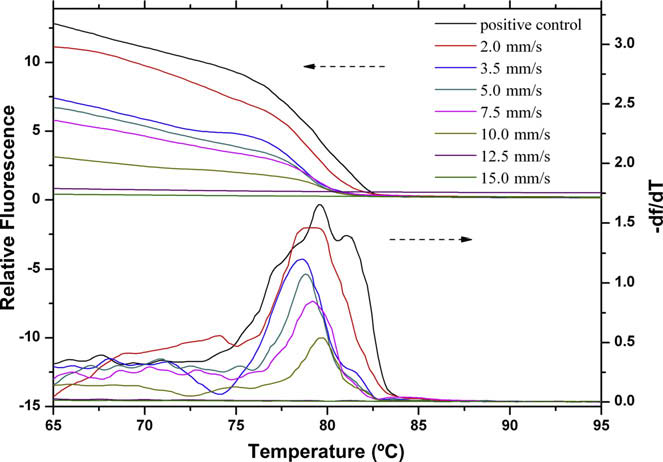
Rapid detection of genetically modified organisms / Y. Li et al. / Anal. Biochem. 385 (2009) 42–49
Fig. 2. Effect of the flow rates on the continuous-flow PCR yield. The top panels show fluorescence images of PCR products of the P35S sequence (A) and the Tnos sequence (B)in 1.5% agarose gel. Lane M: DL 2000 marker; lane 1: positive control PCR product from the conventional PCR machine (a commercial Mastercycler gradient PCR machine);lanes 2 to 8: continuous-flow PCR products at flow rates of 2.0, 3.5, 5.0, 7.5, 10.0, 12.5, and 15.0 mm s1, respectively; lane 9: negative control PCR, PCR mixture solution withno DNA sample run at a flow rate of 5.0 mm s1. The lower panels show a comparison of band intensities of the respective upper panels that was analyzed by image analysissoftware (Quantity One). Values were normalized to the fluorescence of the product from the conventional PCR machine (100%, lane 1).
ities, the amount of PCR products is gradually decreased, but thePCR reaction speed is raised gradually. The corresponding timesof the PCR mixture flowing through the PTFE capillary range fromapproximately 40 to 6 min. At a flow rate of 7.5 mm s1, the PCRproducts can be detected using only approximately 11 min after35 cycles (lane 5 in upper panel of A). In addition, the PCRproducts cannot be easily observed by electrophoresis when theflow rates were increased to 10 mm s1 or higher (lanes 6–8). Thisexperimental phenomenon was also observed while amplifying theTnos sequence of GM soybeans at flow rates ranging from 2.0 to15.0 mm s1 (B, upper and lower panels). This does not nec-essarily mean that there were no products obtained at those rates;more likely, it means that the amount of PCR products under thesePCR conditions was smaller than the detection limit of the fluores-cence scanner associated with the gel imaging system.
However, using the MCA, amplicons can be detected when the
flow velocity was increased to 10 mm s1 In other words,the whole continuous-flow PCR might require 9 min and has a suc-
Fig. 3. Fluorescence melting curves for the amplification products of the Tnos
cessful determination. In addition, MCA requires only approxi-
sequence on the microfluidic device at various flow rates. The various flow rates of the
mately 7 min, which obviates the need to examine PCR products
PCR mixture in the microchannel were 2.0, 3.5, 5.0, 7.5, 10.0, 12.5, and 15.0 mm s1.
on time-consuming agarose gels. After continuous-flow PCR ampli-
The positive control PCR product was from the conventional PCR machine
fication, the LightCycler monitors the decrease of fluorescence
(a commercial Mastercycler gradient PCR machine). After continuous-flow PCR,melting curve analysis was performed with the LightCycler. The products with SYBR
resulting from the release of SYBR Green I during DNA melting
Green I were heated to 95 °C during 15 s, cooled at 60 °C for 20 s, and then slowly
curve analysis by the slow increase of the temperature. Because
heated back to 95 °C at a rate 0.1 °C s1.
the melting curve's shape is dependent on GC content, length,and sequence, the Tm of specific amplicons and unique shape ofthe melting peak can be used to differentiate the target genes
the maximal thermal cycling rate of continuous-flow PCR micro-
and identify them The average Tm (Tm) from three indepen-
fluidics is controlled by the dynamics of Taq DNA polymerase,
dent assays was 78.99 ± 0.24 °C with a coefficient of variation
whose extension rate is approximately 60 to 100 bases s1 at
(CV) of 0.30% for the Tnos sequence (180 bp, G + C 32.8%) and
72 °C In other words, the residence time of PCR mixture
84.60 ± 0.37 °C with a CV of 0.43% for the P35S sequence
in the extension zone was below the kinetic rate of the polymerase
(195 bp, G + C 50.3%). Test results were considered as positive
enzyme In addition, it is also affected by the size of the PTFE
when the Tm was within the Tm ± 3 standard deviations (SD) for
capillary. The thick-walled PTFE capillary possessing low thermal
each sequence The ranges were from 78.27 to 79.71 °C for
conductivity will increase the time required to transfer the heat
Tnos and from 83.49 to 85.71 °C for P35S.
from the outer to the inner of the capillary, and likewise the large
Nevertheless, when the flow rate was raised to 12.5 mm s1 or
diameter of the capillary will reduce the speed of thermal equili-
higher, no signal of PCR products was detected. Maybe it is because
bration of the PCR reaction mixture in the capillary channel. It is
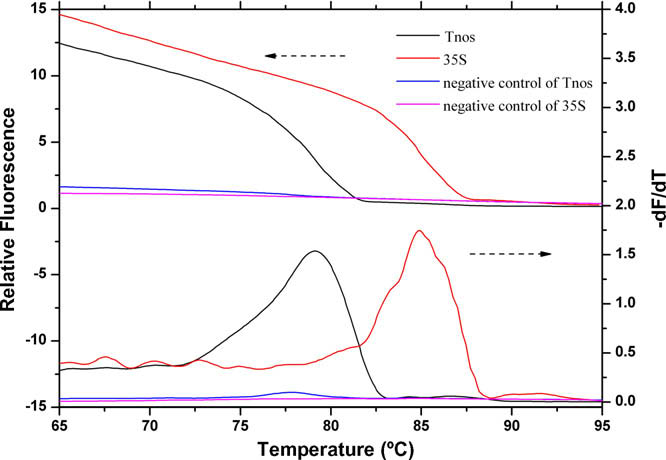
Rapid detection of genetically modified organisms / Y. Li et al. / Anal. Biochem. 385 (2009) 42–49
worth noting that the amount of the products amplified at a flow
electrophoresis detection system is 0.05 ng ll1. The amount of
rate of 2.0 mm s1 is higher than that of the positive control PCR
PCR products under this concentration condition is approximately
products from the conventional PCR machine (lane 2 in upper pa-
10% of the products under a DNA concentration of 7.5 ng ll1.
nel of B). This phenomenon may be attributed to the fact that
Comparing the results of experiments in with those in
the heating and cooling rates of the microfluidics are higher than
, it is not hard to see that the sensitivity of the MCA method
those of the conventional PCR, and so the possibility of nonspecific
is higher than that of agarose gel electrophoresis. Therefore, to fur-
PCR products derived from false priming decreases, thereby
ther enhance the efficiency of amplification, we chose a flow rate of
enhancing the efficiency of the amplification. Moreover, the results
5.0 mm s1 and then used the MCA for the detection of the Tnos
of MCA show that our supposition is reasonable. It is clear that the
sequence, and the sensitivity is improved to 0.005 ng ll1 of the
peak shape of the products amplified on the continuous-flow PCR
DNA concentration There is a weak peak when the DNA
microfluidics is sharper than that of amplicons from the conven-
concentration is reduced to 0.001 ng ll1. However, the signal-
tional PCR machine ().
to-noise ratio is relatively low, so it cannot be considered as a po-
Moreover, to determine that there are no false-positive amplifi-
sitive result. That is, the LOD of the DNA sample that can be used in
cations by this methodology, amplification using 45 cycles was
this continuous-flow PCR device with the MCA detection system is
also performed on this microfluidic device at a flow rate of 5.0
0.005 ng ll1.
On the commercial Mastercycler gradient PCR machine, we also
detected the Tnos sequence to investigate the effect of the amount
Effect of DNA sample concentration on PCR amplification
of initial DNA sample upper and lower panels). Becausethe SVR of the polypropylene tube is relatively small, the possibil-
It is important to study the effect of the amount of initial DNA
ity of adsorption of biomacromolecules onto the tube wall is lower.
sample on continuous-flow PCR amplification. Because the sur-
Therefore, when the DNA concentration is decreased to 0.001 ng
face-to-volume ratio (SVR) of the continuous-flow PCR microflui-
ll1, the PCR product band obtained is also evident.
dics (8 mm1) is much larger than that of the polypropylenetube (1.5 mm1) , there is a greater possibility of adsorption
Successive amplification of different DNA samples
of biomacromolecules onto the capillary inner surface that may in-hibit the PCR amplification. Based on our previous work a
For continuous-flow PCR, one of the important superiorities is
flow rate of 3.5 mm1 was chosen in this set of experiments for
that it is very facile to perform successive DNA amplification by
considering the production and time of amplification. The upper
using a continuous segmented flow of different PCR mixtures con-
panel of A shows the gel electrophoresis results of the Tnos
taining different DNA samples. This format of amplification can
sequence amplified under the DNA sample concentrations from
save time and simplify the operation to a large extent. During re-
7.5 to 0.001 ng ll1, whereas the lower panel shows the relative
cent years, some researchers have studied the successive amplifi-
amounts of obtained PCR products as determined by image analy-
sis software. Values were normalized to the fluorescence of the
contamination-free amplification in a continuous-flow format
products under a DNA sample concentration of 7.5 ng ll1 (100%,
In the current study, we used a method similar to
lane 1). It can be seen from the upper panel of A that the yield
that referred to in Park and coworkers' work to get rid of
of PCR products of the Tnos sequence is decreased with the reduc-
the cross-contamination from successive PCR amplification of the
tion of the DNA sample concentration from 7.5 to 0.001 ng ll1.
Tnos and/or P35S sequences with different samples: GM soybeans,
The lowest DNA concentration that could be visibly detected by
non-GM soybeans, and the negative control. To cleanse the carry-
agarose gel electrophoresis is up to 0.05 ng ll1 (lane 7). Although
over from the preceding segment to the following one, we inter-
no visible product band was apparent for the 0.01-ng ll1 DNA
posed 1 PCR buffer containing 0.4 BPB buffer between each
sample, this does not necessarily mean that no product was gener-
segment. Due to the fact that the buffer is widely used in loading
ated at those concentrations; more likely, it means that the amount
DNA samples on electrophoresis gels, DNA molecules would dis-
of products produced under these PCR conditions was smaller than
solve in it very well. Moreover, it is blue, and so each sample seg-
the detection limit of the fluorescence scanner associated with the
ment is easily located and separated. A illustrates the
gel imaging system. Namely, the limit of detection (LOD) of DNA
segmented-flow mode of continuous PCR amplification of different
sample that can be used in this continuous-flow PCR device with
DNA samples. C demonstrate the successive amplifi-cation of the Tnos and P35S sequences with three segments of PCRmixtures. shows the gel electrophoresis results of succes-sive amplification with six segments of PCR mixtures. As shownin the absence of cross-contamination between samples isdemonstrated, and the injection of negative samples before andafter a positive sample, as shown in confirms that theamplicon was copied from the desired template. The cleaningmethod was able to reduce the PCR residue between amplifica-tions. The experimental results of the experiments indicate thatthere is little carryover and inhibition in this system.
This very high-throughput methodology is nearly qualitative
and determines the presence or absence of GMOs for each of thesamples. It is effectively to control that no such unauthorizedGMO exists in commercialized food. Note that some authorizedGMOs (e.g., Roundup Ready soy) have been adopted by local regu-lations, but there is a positive threshold when there are no safetyissues. The current European Union regulations (1829/2003/ECand 1830/2003/EC) stipulate that the products in which the GMO
Fig. 4. Fluorescence melting curves for the amplification products of the P35S and
contents are more than 0.9% must be labeled. In some cases, the
Tnos sequences on the microfluidic device using 45 cycles at a flow rate of 5.0 mm s1.
optical density in an enzyme-linked immunosorbent assay (ELISA)
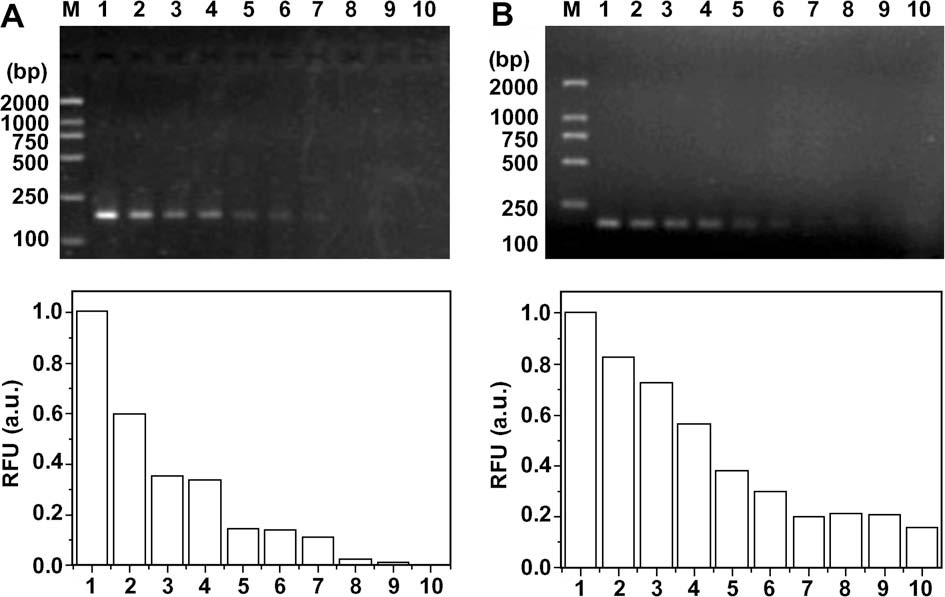
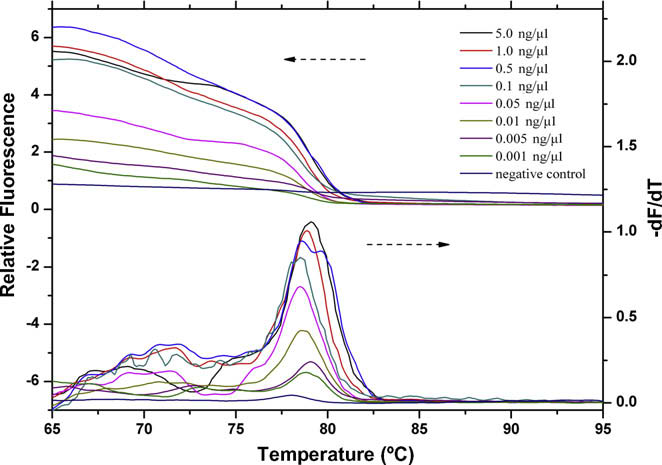
Rapid detection of genetically modified organisms / Y. Li et al. / Anal. Biochem. 385 (2009) 42–49
Fig. 5. Fluorescence intensity of the product gel band as a function of the input DNA molecules. The top panels show the DNA sample concentration effect for theamplification of the Tnos sequence on the continuous-flow PCR microfluidics at a flow rate of 3.5 mm s1 (A) and the same effect on the conventional PCR machine (acommercial Mastercycler gradient PCR machine). (B) Lane M: DL 2000 marker; lanes 1 to 10: PCR products from various concentrations of the input DNA molecules (7.5, 5.0,2.5, 1.0, 0.5, 0.1, 0.05, 0.01, 0.005, and 0.001 ng ll1, respectively). The lower panels show a comparison of band intensities of the respective upper panels that was analyzedby image analysis software (Quantity One). Values were normalized to the fluorescence of the product under a DNA sample concentration of 7.5 ng ll1 (100%, lane 1).
which cannot be discriminated distinctly after the duplex PCRusing agarose gel electrophoresis to identify 15-bp differences. Du-plex PCR assay, which simultaneously amplifies the P35S and Tnossequences in a single PCR, saves significant time and labor com-pared with individual PCR assays. After a careful analysis of therespective experimental Tm, we optimized the concentrations ofprimers to perform the duplex amplification for the P35S and Tnossequences. Each pair of primers was optimized to a final concentra-tion of 200 and 400 nM for the P35S and Tnos sequences, respec-tively. The concentration of Taq DNA polymerase was 0.1 U ll1for the duplex continuous-flow PCR. As shown in , using thatconcentration of Taq DNA polymerase, P35S and Tnos sequenceswere amplified in simplex PCR format respectively and simulta-neously amplified in duplex PCR. It was possible to unambiguouslyamplify and identify each fragment in duplex continuous-flow PCRcoupled with MCA. In addition, in a comparison with , illustrates that the PCR efficiency could be improved with an in-
Fig. 6. Fluorescence melting curves for the amplification products of the Tnos
crease of Taq DNA polymerase concentration .
sequence on the microfluidic device with the different concentrations of the input
The LOD of DNA sample of duplex PCR on this microfluidic de-
DNA molecules. The various concentrations of the input DNA molecules were 5.0,
vice was also assessed. It can be seen from that the sensitivity
1.0, 0.5, 0.1, 0.05, 0.01, 0.005, and 0.001 ng ll1. The negative control PCR was alsoperformed. The amplification was performed at a flow rate of 5.0 mm s1. Other
of duplex PCR detection is estimated to be 0.01 ng ll1. When the
conditions were as in
DNA concentration is reduced to 0.005 ng ll1, there is only a weakpeak of the Tnos sequence but not in the temperature range of the
method, the cycle threshold (Ct) in a real-time PCR (RT–PCR), and
Tm of the P35S sequence.
so on can be calibrated to predict the GMO concentration. Butdoing that rigorously implies good knowledge of the distribution
of the response and its uncertainty. With the method ‘‘quality con-trol by attributes" , this successive amplification in a contin-
The continuous-flow PCR presented here has been shown to be
uous-flow format can also be quantitative.
a new technique for the detection of GMOs. The systems describedare highly convenient for use in routine GMO identification analy-
Duplex continuous-flow PCR systems based on MCA method
sis. The amplification of the P35S and the Tnos sequences could besuccessfully completed within approximately 9 min on this com-
Unlike gel electrophoresis, MCA can distinguish products of the
pact, spiral, channel-based, continuous-flow PCR microfluidics;
same length but different GC/AT ratios. Assuming that Tm differ-
thus, the amplification rate was much faster than that of the con-
ences above 2 °C may allow discrimination of the PCR products,
ventional PCR machine. In addition, the LOD of DNA sample in the
it was easy to differentiate P35S and Tnos sequences with Tm val-
presented continuous-flow PCR microfluidics is estimated to be
ues differing by approximately 5.6 °C. So, we chose this method for
0.005 ng ll1. Furthermore, duplex continuous-flow PCR was also
the postamplification detection of the P35S and Tnos sequences,
reported for the detection of the P35 and Tnos sequences simulta-
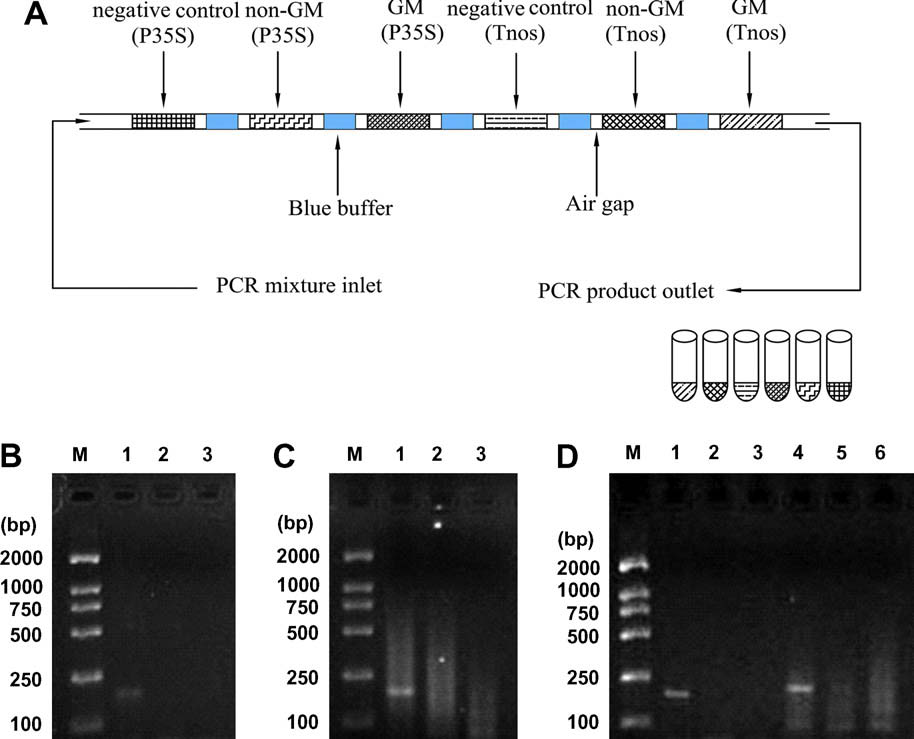
Rapid detection of genetically modified organisms / Y. Li et al. / Anal. Biochem. 385 (2009) 42–49
Fig. 7. (A) Segmented-flow mode of continuous PCR amplification of different DNA samples. (B) Successive amplification of Tnos sequence with different DNA samples: GMsoybeans, non-GM soybeans, and negative control. (C) Successive amplification of P35S sequence with different DNA samples. (D) Successive amplification of Tnos and P35Ssequences with different DNA samples. Lane M: DL 2000 marker; lanes 1 to 3 in panels B and D: sequential amplification of Tnos sequence with different DNA samples: GMsoybeans, non-GM soybeans, and negative control, respectively; lanes 1 to 3 in panel C and lanes 4 to 6 in panel D: sequential amplification of P35S sequence with differentDNA samples: GM soybeans, non-GM soybeans, and negative control, respectively.
Fig. 8. Melting curve analysis of duplex PCR products on the microfluidic device.
Fig. 9. Fluorescence melting curves for duplex PCR products of the microfluidicdevice with the different concentrations of the input DNA molecules. The various
The amplification was performed at a flow rate of 5.0 mm s1. Other conditionswere as in Fig. 3.
concentrations of the input DNA molecules were 5.0, 1.0, 0.5, 0.1, 0.05, 0.01, and0.005 ng ll1. The amplification was performed at a flow rate of 5.0 mm s1.
neously coupled with MCA of the products, and the LOD of DNA
is time-consuming, is poorly portable, and requires multiple labo-
sample is assessed to be 0.01 ng ll1. It should be noted that with
ratory instruments. However, it still takes a long time to perform
continuous-flow PCR it could be very easy to perform successive
on-line sample preparation on the PCR microfluidics. Integration
DNA amplification, which could save time and simplify the opera-
with analytical detection, such as capillary electrophoresis fol-
tion to a large extent.
lowed by laser-induced fluorescence detection, electrochemilumi-
This work represents the first step for an automated continu-
nescent detection, DNA microarray hybridization, and so on could
ous-flow PCR system for the detection of GMOs, and several further
decrease analytical cost, enhance sensitivity and speed of detec-
improvements still are required for the automated rapid detection.
tion, and effectively overcome the errors resulting from some man-
Those include integration with preamplification processes, such as
ual operations. Our current efforts are focused on off-line and on-
DNA extraction and sample mixing, and postamplification product
line electrochemiluminescent detection for detecting GMOs
detection. On-line sample preparation is superior in speed and
on this continuous-flow PCR microfluidics. Although the
sample consumption to off-line manual sample preparation, which
PCR microfluidics provides many advantages over the conventional
Rapid detection of genetically modified organisms / Y. Li et al. / Anal. Biochem. 385 (2009) 42–49
PCR device, the miniaturization also raises some challenging issues
[23] B.C. Giordano, J. Ferrance, S. Swedberg, A.F.R. Hühmer, J.P. Landers, Polymerase
such as the adsorption of the reagents to the channel surface, the
chain reaction in polymeric microchips: DNA amplification in less than240 seconds, Anal. Biochem. 291 (2001) 124–132.
proneness to evaporation of the sample solution and formation
[24] J. Liu, M. Enzelberger, S. Quake, A nanoliter rotary device for polymerase chain
of gas bubbles, the requirement of precise temperature control,
reaction, Electrophoresis 23 (2002) 1531–1536.
and so on. Despite these obstacles, the potential of PCR microflui-
[25] C.S. Zhang, J.L. Xu, J.Q. Wang, H.P. Wang, Experimental study of continuous-
dics as a future nucleic acid amplification is still attractive.
polytetrafluoroethylene capillary, Chin. J. Anal. Chem. 34 (2006) 1197–1202.
[26] P.A. Auroux, Y. Koc, A. deMello, A. Manz, P.J. Day, Miniaturized nucleic acid
analysis, Lab Chip 4 (2004) 534–546.
[27] J. West, M. Becker, S. Tombrink, A. Manz, Micro total analysis systems: latest
achievements, Anal. Chem. 80 (2008) 4403–4419.
This research was supported by the National Natural Science
[28] M.U. Kopp, A.J. de Mello, A. Manz, Chemical amplification: continuous-flow
Foundation of China (30700155 and 30600128), the National High
PCR on a chip, Science 280 (1998) 1046–1048.
[29] K. Sun, A. Yamaguchi, Y. Ishida, S. Matsuo, H. Misawa, A heater-integrated
Technology Research and Development Program of China (863 Pro-
transparent microchannel chip for continuous-flow PCR, Sens. Actuat. B 84
gram) (2007AA10Z204), and the Natural Science Foundation of
(2002) 283–289.
Guangdong Province (7005825).
[30] P.J. Obeid, T.K. Christopoulos, H.J. Crabtree, C.J. Backhouse, Microfabricated
device for DNA and RNA amplification by continuous-flow polymerase chainreaction and reverse transcription–polymerase chain reaction with cycle
number selection, Anal. Chem. 75 (2003) 288–295.
[31] J.H. Liu, X.F. Yin, G.M. Xu, Z.L. Fang, H.Z. Chen, Studies on a microfluidic chip
[1] E. Gachet, G.G. Martin, F. Vigneau, G. Meyer, Detection of genetically modified
based on the continuous flow PCR amplification system, Chem. J. Chin. Univ.
organisms (GMOs) by PCR: a brief review of methodologies available, Trends
24 (2003) 232–235.
Food Sci. Technol. 9 (1999) 380–388.
[32] L.Y. Yao, B.A. Liu, T. Chen, S.B. Liu, T.C. Zuo, Micro flow-through PCR in a PMMA
[2] A. Nadal, A. Coll, J.L. La Paz, T. Esteve, M. Pla, A new PCR–CGE (size and color)
chip fabricated by KrF excimer laser, Biomed. Microdevices 7 (2005) 253–257.
method for simultaneous detection of genetically modified maize events,
[33] J.A. Kim, J.Y. Lee, S. Seong, S.H. Cha, S.H. Lee, J.J. Kim, T.H. Park, Fabrication and
Electrophoresis 27 (2006) 3879–3888.
characterization of a PDMS–glass hybrid continuous-flow PCR chip, Biochem.
[3] D. Rodríguez-Lázaro, B. Lombard, H. Smith, A. Rzezutka, M. D'Agostino, R.
Eng. J. 29 (2006) 91–97.
Helmuth, A. Schroeter, B. Malorny, A. Miko, B. Guerra, J. Davison, A. Kobilinsky,
[34] M. Hashimoto, F. Barany, F. Xu, S.A. Soper, Serial processing of biological
M. Hernández, Y. Bertheau, N. Cook, Trends in analytical methodology in food
reactions using flow-through microfluidic devices: coupled PCR/LDR for the
safety and quality: monitoring microorganisms and genetically modified
detection of low-abundant DNA point mutations, Analyst 132 (2007) 913–
organisms, Trends Food Sci. Technol. 18 (2007) 306–319.
[4] T. Abdullah, S. Radu, Z. Hassan, J.K. Hashim, Detection of genetically modified
[35] M. Curcio, J. Roeraade, Continuous segmented-flow polymerase chain reaction for
soy in processed foods sold commercially in Malaysia by PCR-based method,
high-throughput miniaturized DNA amplification, Anal. Chem. 75 (2003) 1–7.
Food Chem. 98 (2006) 575–579.
[36] N. Park, S. Kim, J.H. Hahn, Cylindrical compact thermal-cycling device for
[5] A. Holst-Jensen, GMO detection methods and validation review, National
continuous-flow polymerase chain reaction, Anal. Chem. 75 (2003) 6029–
Veterinary Institute, Section of Food & Feed Microbiology, Oslo, Norway, 2001.
[6] G. Ujhelyi, B. Vajda, E. Béki, K. Neszlényi, J. Jakab, A. Jánaosi, E. Némedi, E.
[37] M. Hashimoto, P.C. Chen, M.W. Mitchell, D.E. Nikitopoulos, S.A. Soper, M.C.
Gelencsér, Surveying the RR soy content of commercially available food
Murphy, Rapid PCR in a continuous flow device, Lab Chip 4 (2004) 638–645.
products in Hungary, Food Control 19 (2008) 967–973.
[38] J.H. Liu, X.F. Yin, Z.L. Fang, Automatic continuous amplification of long
[7] R.K. Saiki, S. Scharf, F. Faloona, K.B. Mullis, G.T. Horn, H.A. Erlich, N.
fragments DNA with spiral flow through PCR microchip, Chem. J. Chin. Univ.
Arnheim, Enzymatic amplification of b-globin genomic sequences and
25 (2004) 30–34.
restriction site analysis for diagnosis of sickle cell anemia, Science 230
[39] K.D. Dorfman, M. Chabert, J.H. Codarbox, G. Rousseau, P. de Cremoux, J.L.
(1985) 1350–1354.
Viovy, Contamination-free continuous flow microfluidic polymerase chain
[8] T.H. Varzakas, G. Chryssochoidis, D. Argyropoulos, Approaches in the risk
reaction for quantitative and clinical applications, Anal. Chem. 77 (2005)
assessment of genetically modified foods by the Hellenic Food Safety
Authority, Food Chem. Toxicol. 45 (2007) 530–542.
[40] C.S. Zhang, J.L. Xu, J.Q. Wang, H.P. Wang, Continuous-flow polymerase chain
[9] J.F. Liu, D. Xing, X.Y. Shen, D.B. Zhu, Detection of genetically modified
reaction microfluidics by using spiral capillary channel embedded on copper,
organisms by electrochemiluminescence PCR method, Biosens. Bioelectron.
Anal. Lett. 40 (2007) 497–511.
20 (2004) 436–441.
[41] C.S. Zhang, D. Xing, J.L. Xu, Continuous-flow PCR microfluidics for rapid DNA
[10] K. Cankar, V. Chauvensy-Ancel, M.N. Fortabat, K. Gruden, A. Kobilinsky, J. Zel, Y.
amplification using thin film heater with low thermal mass, Anal. Lett. 40
Bertheau, Detection of nonauthorized genetically modified organisms using
(2007) 1672–1685.
differential quantitative polymerase chain reaction: application to 35S in
[42] J. Chiou, P. Matsudaira, A. Sonin, D. Ehrlich, A closed-cycle capillary
maize, Anal. Biochem. 376 (2008) 189–199.
polymerase chain reaction machine, Anal. Chem. 73 (2001) 2018–2021.
[11] J.F. Liu, D. Xing, X.Y. Shen, D.B. Zhu, Electrochemiluminescence polymerase
[43] P. Belgrader, C.J. Elkin, S.B. Brown, S.N. Nasarabadi, R.G. Langlois, F.P.
chain reaction detection of genetically modified organisms, Anal. Chim. Acta
Milanovich, B.W. Colston, G.D. Marshall Jr., A reusable flow-through
537 (2005) 119–123.
polymerase chain reaction instrument for the continuous monitoring of
[12] A.J. de Mello, DNA amplification: does ‘‘small" really mean ‘‘efficient"?, Lab
infectious biological agents, Anal. Chem. 75 (2003) 3446–3450.
Chip 1 (2001) 24–29.
[44] W. Wang, Z.X. Li, R. Luo, S.H. Lü, A.D. Xu, Y.J. Yang, Droplet-based micro
[13] C.S. Zhang, J.L. Xu, The design development of continuous-flow polymerase
oscillating-flow PCR chip, J. Micromech. Microeng. 15 (2005) 1369–1377.
chain reaction chip, Chin. J. Anal. Chem. 33 (2005) 729–734.
[45] J.Y. Cheng, C.J. Hsieh, Y.C. Chuang, J.R. Hsieh, Performing microchannel
[14] I. Schneegaß, J.M. Kohler, Flow-through polymerase chain reactions in chip
temperature cycling reactions using reciprocating reagent shuttling along a
thermocyclers, Rev. Mol. Biotechnol. 82 (2001) 101–121.
radial temperature gradient, Analyst 130 (2005) 931–940.
[15] H. Nagai, Y. Murakami, K. Yokoyama, E. Tamiya, High-throughput PCR in
[46] M. Hernández, D. Rodríguez-Lázaro, T. Esteve, S. Prat, M. Pla, Development of
silicon based microchamber array, Biosens. Bioelectron. 16 (2001) 1015–1019.
melting temperature-based SYBR Green I polymerase chain reaction methods
[16] M. A. Northrup, M. T. Ching, R. M. White, R. T. Watson, DNA amplification in a
for multiplex genetically modified organism detection, Anal. Biochem. 323
microfabricated reaction chamber, in: proceedings of the 7th International
(2003) 164–170.
Conference on Solid State Sensors and Actuators, Yokohama, Japan, 1993, pp.
[47] R.H. Lekanne Deprez, A.C. Fijnvandraat, J.M. Ruijter, A.F.M. Moorman,
Sensitivity and accuracy of quantitative real-time polymerase chain reaction
[17] H. Nakano, K. Matsuda, M. Yohda, T. Nagamune, I. Endo, T. Yamane, High speed
using SYBR Green I depends on cDNA synthesis conditions, Anal. Biochem. 307
polymerase chain reaction in constant flow, Biosci. Biotechnol. Biochem. 58
(2002) 63–69.
(1994) 349–352.
[48] K.M. Ririe, R.P. Rasmussen, C.T. Wittwer, Product differentiation by analysis of
[18] C.S. Zhang, J.L. Xu, W.L. Zheng, PCR microfluidic devices for DNA amplification,
DNA melting curves during the polymerase chain reaction, Anal. Biochem. 245
Biotechnol. Adv. 24 (2006) 243–284.
(1997) 154–160.
[19] C.S. Zhang, D. Xing, Miniaturized PCR chips for nucleic acid amplification and
[49] K.S. Elenitoba-Johnson, S.D. Bohling, C.T. Wittwer, T.C. King, Multiplex PCR by
analysis: latest advances and future trends, Nucleic Acids Res. 35 (2007) 4223–
multicolor fluorimetry and fluorescence melting curve analysis, Nat. Med. 2
(2001) 249–253.
[20] C.S. Zhang, D. Xing, Y.Y. Li, Micropumps, microvalves, and micromixers within
[50] W. Fan, T. Hamilton, S. Webster-Sesay, M.P. Nikolich, L.E. Lindler, Multiplex
PCR microfluidic chips: advances and trends, Biotechnol. Adv. 25 (2007) 483–514.
real-time SYBR Green I PCR assay for detection of tetracycline efflux genes of
[21] J.G. Lee, K.H. Cheong, N. Huh, S. Kim, J.W. Choi, C. Ko, Microchip-based one step
gram-negative bacteria, Mol. Cell Probes 21 (2007) 245–256.
DNA extraction and real-time PCR in one chamber for rapid pathogen
[51] K.M. Remund, D.A. Dixon, D.L. Wright, L.R. Holden, Statistical considerations in
identification, Lab Chip 6 (2006) 886–895.
seed purity testing for transgenic traits, Seed Sci. Res. 11 (2001) 101–119.
[22] R. Prakash, K.V.I.S. Kaler, An integrated genetic analysis microfluidic platform
[52] A. Kobilinsky, Y. Bertheau, Minimum cost acceptance sampling plans for grain
with valves and a PCR chip reusability method to avoid contamination,
control, with application to GMO detection, Chemometr. Intell. Lab. 75 (2005)
Microfluid Nanofluid 3 (2007) 177–187.
Source: http://chglib.icp.ac.ru/subjex/2011/pdf/Anal.Biochem/Analytical%20Biochem.%202009,%20vol.%20385,%20n1%20p.%2042-49.PDF
CHILDREN EXCLUDED CHILDREN EXCLUDED CHILDREN EXCLUDED CHILDREN EXCLUDED HIgH DRUg PRICES HIgH DRUg PRICES HIgH DRUg PRICES HIgH DRUg PRICES HIgH DRUg PRICES g RESTRICTED Report of the Inquiry of the All Party Parliamentary Group on HIV and AIDS into access to medicines in the developing world DENIED f f EXCLUDED
Guide to yourColonoscopy or Upper GI Endoscopy Pre-Admission Phone Interview Date & Time: (you will be given the time of your procedure on this call) Date of Procedure: _ Your Upcoming Colonoscopy or Upper GI Endoscopy At Grand Itasca, we want to make sure that your endoscopy is as pleasant as possible. This guide is designed to answer any questions