Investigation of selected signalling system genes in pathological gambling finalreport
Investigation of Selected Signalling System Genes in Pathological Gambling
Final Report
Principal Investigator and the Project Contact Person:
James L. Kennedy, M.D., Director, Neuroscience Research, CAMH
250 College St., R-31 – Toronto, ON – M5T 1R8 – Phone: 416- 979-4987
FAX: 416- 979-4666.
[email protected]
Principal Co-Investigator:
Daniela S. S. Lobo, M.D., Ph.D., Neurogenetics, CAMH
250 College St., R-32 – Toronto, ON – M5T 1R8 – Phone: 416- 535-8501 extension
FAX: 416- 979-4666.
[email protected]
Table of Contents:
Abstract
1. Introduction
1.1 Literature Review
2. Research Design and Methodological Approach
2.2 Gene and Polymorphism (SNP) Selection
2.3 Statistical Analysis
2.4 Sequencing of DRD4 exon III region
3. Results
3.1 PG subjects x Control Subjects
3.2 PG subjects with alcohol and/or drug abuse
x Control Subjects
3.3 PG subjects with alcohol and/or drug abuse
– PG-ADA (n =99) x PG subjects without
3.4. DRD4 sequencing analysis
4. Limitations and Changes to Original Proposal
5. Discussion
6. References
List of Tables
List of genes and SNPs selected for genotyping
within their respective chromosomes
Haplotype comparisons between problem
gamblers (PG) with healthy control subjects
Haplotype comparisons between problem
gamblers with alcohol and/or drug abuse (PG-
ADA) with control subjects
Haplotype comparisons between problem
gamblers with alcohol and/or drug abuse (PG-
ADA) with PG subjects without substance abuse
List of Figures
Explanation of symbols in figures 1-5
Figure 1: Neuroactive ligand receptor interaction pathway
Figure 2: Long-term potentiation pathway
Figure 3: GnRH signalling pathway
Figure 4: MAPK signalling pathway
Figure 5: Gap junction pathway
Figure 6: Diagrammatic Representation of the DRD4 gene and DRD4 exon III VNTR
Figure 7: Dopamine D4 receptor graphical representation
Figure 8: Screenshot of the sequence alignment (DRD4 exon III)
Figure 9: Screenshot of the deletion on DRD4 exon III from one subject in our sample
We would like to thank Sajid Shaik for his contribution in the preparation of DNA samples and
coordination of genotyping. We would like to thank Maria Tampakeras and Natalie Freeman for
sequencing and analyzing our data on DRD4. As always, we are grateful for all the contribution
provided by our research subjects.
We would like to thank OPGRC for its continued support in funding our project.
Abstract:
Background: Several studies have provided evidence for a biological basis for problem gambling
(PG): first-degree relatives (FDR) of PG subjects present a higher than expected chance to develop PG
and a large twin study has reported that genetic factors account for 52% of the variance of PG
diagnosis. The increased risk for developing substance addiction in FDR of PG subjects, the high
comorbidity between substance dependence and PG, and a common underlying genetic vulnerability
for PG and alcohol dependence provide converging evidence that a common neurobiological system
underlies both substance addiction and PG.
Objectives: 1. to continue the investigation of the genetic
basis of problem gambling (PG), through the analysis of genes involved in brain's signalling system
that underlies addictive disorders; 2. to further investigate how the variations in the sequence of the 2
and 7-repeat alleles of the D4 receptor gene (DRD4) are associated with PG severity (from our
previous results on the association of DRD4 and PG).
Methods: DNAs and clinical data from 514 PG
subjects and 214 controls assessed during our previously funded OPGRC projects.
Hypotheses: 1.
genes underlying common genetic pathways in substance addiction will also be associated with
increased vulnerability for PG; 2. variations in the sequence of the 2 and 7-repeat alleles of the D4
receptor gene will better predict PG severity.
Gene selection: 1. based on our previous association of
DRD4 2 and 7-repeat alleles with PG severity, we will proceed to sequence these gene variants; 2.
addiction-related genes were selected through the Knowledgebase for Addiction Related Genes; 3.
variants within each gene were selected using
tag SNPs in order to provide maximum gene coverage.
Statistical analysis: Three groups were used for analysis: 1. PG subjects x Control subjects; 2.PG
subjects with alcohol and/or drug abuse x PG subjects without history of substance misuse and PG
subjects with substance misuse compared with healthy control subjects. Genetic analysis was
performed for haplotypes (blocks of variants) using modified qui-squared tests implemented in the
software Golden Helix SNP & variation suite version 7.Correction for multiple testing was performed
through False Discovery Rate tests (FDR).
Results: Haplotypes in CAMK2D, HTR2A, PRKACB, and
PLD2 were significantly associated with PG. No significant associations were found in the comparison
between PG with alcohol and/or drug abuse and controls, and between PG without substance abuse and
PG with alcohol and/or drug abuse. Nominal associations were found with CAMK2D, dopamine D2,
serotonin 2A, and glutamate receptor genes. Sequencing of DRD4 exon III VNTR revealed a new
deletion on the 7-repeat allele sequence that may alter gene expression. Our associations suggest that,
similarly to substance addiction, PG is associated with genes involved in neuronal plasticity
(CAMK2D), signal transduction (PRKCAB), and serotonin signalling (HTR2A). PLD2A is regulated
by G-coupled receptors (dopamine, glutamate), suggesting that altered dopamine release reported in PG
may be linked to down-stream alterations in dopamine signalling pathways. Our findings corroborate
the view of PG as an addiction and suggest that further investigation of these signalling pathways
should provide a better understanding of the neurobiology of PG.
Application of findings: Our results
have provided further insight in the neurobiological processes underlying PG. The reported
associations with genes that synthesize protein kinases (CAMK2A, PRKCAB) open the possibility of
testing protein-kinases inhibitors for the treatment of PG.
Key words:pathological gambling, problem gambling, genetics, addiction
• Goal #1: To continue the investigation of the genetic basis of problem gambling (PG), through the analysis of genes involved in brain's signalling systems that underlie addictive disorders, building on
our readily available sample of DNAs from PG subjects and controls and on the newly available
technology and evidence.
• Goal #2: In our previous research we have found that the rare 7-repeat allele of the dopamine receptor type 4 gene polymorphism (DRD4 exon III VNTR) was significantly associated with PG and that the
2-repeat allele of DRD4 exon III VNTR showed a trend of association with PG. It is known that there
is variation within the sequence that composes the 2- and 7-repeat alleles, but there has been little
research on how these variations might impact associations of these alleles to complex traits (e.g.,
Novelty Seeking, PG). Our objective is to further investigate if the variations in the sequence of the 2
and 7-repeat alleles of the D4 receptor gene are associated with PG severity.
1.1 Literature Review:
Pathological gambling is defined as a persistent and recurrent maladaptive behaviour that disrupts
personal, professional, and family relationships and is not better explained by a manic episode (DSM-
IV, APA). Evidence from several studies suggests that PG presents a biological basis. Family studies
have shown that first-degree relatives (FDR) of PG subjects present a higher than expected chance to
develop PG and a large twin study has reported that the
genetic factors account for approximately
50% of the variance of PG diagnosis in men (Eisen, et al., 1998; Eisen, et al., 2001)
and women
(Slutske, Zhu, Meier, & Martin, 2010).
Since Marks (Marks, 1990) proposed that PG should be considered as an addictive disorder (i.e. as a
behavioural addiction), studies have investigated the relationship between substance and behavioural
addictions (Blanco, Moreyra, Nunes, Saiz-Ruiz, & Ibanez, 2001; Cardinal & Everitt, 2004). Currently,
several lines of investigation provide converging evidence that a common neurobiological system
underlies both substance addiction and PG:
1. Lifetime Comorbidity: Individuals diagnosed as pathological gamblers present a significantly higher
risk to develop (at any point in life) substance dependence compared to the general population (Black
& Moyer, 1998; Bland, Newman, Orn, & Stebelsky, 1993; Crockford & el-Guebaly, 1998;
Cunningham-Williams, Cottler, Compton, & Spitznagel, 1998; Lesieur & Heineman, 1988; Lynch,
Maciejewski, & Potenza, 2004; Maccallum & Blaszczynski, 2002; Petry, Stinson, & Grant, 2005;
Ross, Glaser, & Germanson, 1988; Roy, et al., 1988; Vitaro, Brendgen, Ladouceur, & Tremblay,
2001). Likewise, a person who had a diagnosis of substance dependence (at any point in life) has a
greater risk of being diagnosed with PG (Daghestani, Elenz, & Crayton, 1996; Feigelman, Wallisch, &
Lesieur, 1998; Lesieur, Blume, & Zoppa, 1986; Petry, 2001; Ross, et al., 1988; Shaffer & Korn, 2002;
Spunt, Lesieur, Hunt, & Cahill, 1995; Welte, Barnes, Wieczorek, Tidwell, & Parker, 2001). For
instance, approximately 70% of PG subjects present nicotine dependence (Crockford & el-Guebaly,
1998) and 50 to 70% present alcohol abuse or dependence (McCormick, Russo, Ramirez, & Taber,
1984; Petry, et al., 2005). Pathological gambling rates has also been reported to be increased among
subjects under methadone maintenance (7 – 52%) (Feigelman, Kleinman, Lesieur, Millman, & Lesser,
1995; Ledgerwood & Downey, 2002; Spunt, 2002; Spunt, et al., 1995; Weinstock, Blanco, & Petry,
2006), and among cocaine dependent subjects (8 – 12%) (Hall, et al., 2000; Kausch, 2003; Toneatto &
Brennan, 2002).
2 Family History: FDR of PG subjects have significantly increased risk to develop substance
dependence (Jacobs, 1989; Ramirez, McCormick, Russo, & Taber, 1984; Roy, et al., 1988) compared
to the general population. FDR of substance dependent individuals are also at a higher than expected
risk to develop PG (Herzog, Keller, Lavori, Kenny, & Sacks, 1992; Jacobs, 1989; Lesieur & Heineman,
1988; Ramirez, et al., 1984).
3 Genetics Research: In general, twin studies have provided evidence of a shared common
vulnerability for any addiction, regardless of the substance used (Karkowski, Prescott, & Kendler,
2000; Kendler, Jacobson, Prescott, & Neale, 2003; Tsuang, et al., 1998). PG is reported to share 12 to
20% of its genetic risk with the risk for alcohol dependence (Slutske, et al., 2000), and research suggest
that this shared vulnerability extends to the pathological use of natural rewards (i.e., food and sex) and
to substance dependence (Kelley, 1999; Pelchat, 2002; Shaffer, et al., 2004).
4 Clinical Research: Clinical studies have shown that opioid antagonists, such as naltrexone and
nalmefene, can be effective in the treatment of both PG (Grant, Kim, & Hartman, 2008; Grant, et al.,
2006; Kim, Grant, Adson, & Shin, 2001) and alcohol dependence (Mason, Salvato, Williams, Ritvo, &
Cutler, 1999; Volpicelli, Alterman, Hayashida, & O'Brien, 1992), suggesting that a common biological
pathway is involved in the response to these medications in both conditions.
The dopaminergic system has a well established role in the development of drug priming through the
release of dopamine in the brain's reward system, more specifically in the nucleus accumbens (Kalivas
1 Please note that the dopaminergic system closely interacts with other neurotransmitter systems also involved in substance addiction (glutamate, serotonin), which will not be described here in respect to space limitations.
& Volkow, 2005). The nucleus accumbens works in close relationship with other areas of the brain that
compose the brain's reward system: ventral tegmental area, prefrontal cortex, locus coeruleous,
amygdala, and hippocampus. Research studies have implicated also the brain's reward system in the
pathophysiology of behavioural addictions (Chambers & Potenza, 2003; Chambers, Taylor, & Potenza,
2003; Potenza, 2001). Imaging studies on PG subjects have shown that: 1. when exposed to gambling-
related cues, PG subjects (but not controls) present increased activation of the dorsolateral pre-frontal
cortex (DLPFC) (Crockford, Goodyear, Edwards, Quickfall, & el-Guebaly, 2005) and a relatively
decreased activity in brain regions implicated in impulse regulation (frontal and orbitofrontal cortex,
caudate/basal ganglia, and thalamus) compared with controls (Potenza, et al., 2003); 2. PG is related to
response perseveration and diminished reward and punishment sensitivity as indicated by
hypoactivation of the ventrolateral prefrontal cortex (VLPFC) when money is gained and lost (de
Ruiter, et al., 2009); as well as to heightened limbic and sensory activation when betting for money
(Hollander, et al., 2005). In summary, research has shown that an overlap exists between brain regions
associated with substance addiction and those associated with PG.
Throughout the years, researchers have described different signalling pathways involved in substance
addiction. For instance, the MAPK signalling pathway has been suggested to have a role in regulating
synaptic plasticity related to long-lasting changes in memory associated with substance addiction
(Wang, Fibuch, & Mao, 2007), and the long-term potentiation pathway has been linked to adaptations
in glutamatergic transmission and synapse plasticity induced by substance addiction (Jones & Bonci,
2005). Recently, a group of researchers has integrated all the available research data (between 1976 and
2006) regarding genetic and biological pathways in addiction and developed a database of addiction
related genes placed within five common signalling pathways for substance addiction (C. Y. Li, Mao,
& Wei, 2008). Below are brief descriptions of each pathway. Figures 1-5 provide a diagrammatic
representation of each pathway. It is important to note that these pathways are not isolated and
members (genes, proteins) of one pathway may also play a role in another pathway.
A. Neuroactive-ligand receptor interaction pathway:
The neuroactive-ligand pathway is one of the pathways involved in processing information from the
environment through signalling molecules, such as neurotransmitters. G-coupled protein receptors,
such as dopamine and metabotrobic glutamate family receptors, are part of this pathway. Both
dopamine receptors and genes, and metabotropic glutamate receptors have been significantly associated
with addictions. Several other peptides, proteins, and hormones are part of this pathway.
B. Long-term potentiation:
Long-term potentiation is a pathway specific to the nervous system. Hippocampal long-term
potentiation (LTP), a
long-lasting increase in synaptic efficacy, is the molecular basis for learning
and memory. Stimulation of neuronal afferents in the hippocampus induces glutamate release and
activation of glutamate receptors in neuronal dendrites. A large increase in Ca2+ intake resulting from
influx through NMDA receptors leads to constitutive activation of CaM kinase II (CaMKII).
It is
hypothesized that postsynaptic Ca2+ increases generated through NMDA receptors activate several
signal transduction pathways including the MAPK and cAMP regulatory pathways.
C. Gonadotropin Releasing Hormone (GnRH) signalling pathway:
The GnRH pathway is an endocrine pathway involved in hormone secretion. Gonadotropin-releasing
hormone (GnRH) secretion from the hypothalamus acts upon its receptor in the anterior pituitary to
regulate the production and release of the gonadotropins, LH and FSH. The GnRHreceptor is coupled
to proteins that activate phospholipase C which transmits its signal to diacylglycerol (DAG) and
inositol 1,4,5-trisphosphate (IP3). DAG activates the intracellular protein kinase C (PKC) pathway and
IP3
stimulates release of intracellular calcium.
Signalling downstream of protein kinase C (PKC)
leads to transactivation of the epidermal growth factor (EGF) receptor and activation of mitogen-
activated protein kinases (MAPKs). Active MAPKs translocate to the nucleus, resulting in activation
of transcription factors and rapid induction of genes.
D. Mitogen-activated protein kinase (MAPK) signalling pathway:
The MAPK pathway is also one of the pathways involved in processing information from the
environment through signal transduction, by regulating neuronal plasticity associated with memory
function and addictive properties of substances. The MAPK cascade is a highly conserved module that
is involved in various cellular functions in various animal species, including cell proliferation,
differentiation and migration. Mammals express at least four distinctly regulated groups of MAPKs,
extracellular signal-related kinases (ERK)-1/2, Jun amino-terminal kinases (JNK1/2/3), p38 proteins
(p38alpha/beta/gamma/delta) and ERK5 that are activated by specific MAPK-kinases (MAPKK):
MEK1/2 for ERK1/2, MKK3/6 for the p38, MKK4/7 (JNKK1/2) for the JNKs, and MEK5 for ERK5.
Each MAPK kinase, however, can be activated by more than one MAPK kinase-kinase (a kinase that is
activated by another kinase), increasing the complexity and diversity of MAPK signalling. Presumably
each MAPKKK confers responsiveness to distinct stimuli.
E. Gap junction pathway:
The Gap junction pathway is involved in cell (including neuronal) communication. Gap junctions
contain intercellular channels that allow direct communication between the cytosolic compartments of
adjacent cells. Each gap junction channel is formed by docking of two 'hemichannels', each containing
six connexins, contributed by each neighbouring cell. These channels permit the direct transfer of small
molecules including ions, amino acids, nucleotides, second messengers and other metabolites between
adjacent cells. Gap junctional communication is essential for many physiological events, including
embryonic development, electrical coupling, metabolic transport, apoptosis, and tissue homeostasis.
Communication through Gap Junction is sensitive to a variety of stimuli, including changes in the level
of
intracellular Ca2+, pH, transjunctional applied voltage and phosphorylation/ dephosphorylation
As it can be inferred from the descriptions above, these pathways have points at which they merge or
interact with one another, at times sharing gene products (proteins, enzymes, hormones) and at times
activating another pathway. Through the construction of the KARG database, it was observed that
positive feedback loops interlinked the pathways with each other through CAMKII. Two of these
positive feedback loops involved signal transduction and would be considered ‘‘fast'' loops, whereas
the other two loops involved transcription and translation and would be considered ‘‘slow'' loops (C.
Y. Li, et al., 2008). Previous research had found that coupled fast and slow positive feedback loops
could create a switch that was inducible and resistant to noise and played key roles in discontinuous
and irreversible biological process, features characteristic of addiction (Abrieu, Doree, & Fisher, 2001;
Brandman, Ferrell, Li, & Meyer, 2005). Activation of CAMKII has also been reported to play
fundamental roles in the development and maintenance of addiction states (Noda & Nabeshima, 2004;
Tang, Shukla, Wang, & Wang, 2006). Disruption of CaMKII translation in neurons (dendrites)
impaired the stabilization of synaptic plasticity and memory consolidation (Miller, et al., 2002; Valjent,
Corbille, Bertran-Gonzalez, Herve, & Girault, 2006). Taking this evidence together, the KERG
database authors have suggested that the fast and slow positive feedback loops interlinked through
CAMKII may be essential for the development and consolidation of addiction and may provide a
systems-level explanation for some of the characteristics of addictive disorders (C. Y. Li, et al., 2008).
In summary, based on research evidence suggesting common biological pathways for substance
addictions and PG and on the recently compiled database of addiction related genetic pathways
(KARG), we
have hypothesized that genes underlying signalling pathways in substance addiction
will also be associated with increased vulnerability for PG. More specifically, we expect that genes
located on chromosomes 4, 5, 9, 10, 11 and 17 will be associated with PG, since these chromosomes
have the strongest evidence for harbouring susceptibility genes for addictions (M. D. Li & Burmeister,
Aside from investigating the aforementioned hypothesis, we have also proposed to investigate whether
the variations in the sequence of the 2 and 7-repeat alleles of the D4 receptor gene (DRD4 exon III
VNTR – see Figure 6) are associated with PG severity. This investigation is based on our previous
results showing that DRD4 (exonIII) 7-repeat allele is associated with severity of PG, and that this
association is not moderated by sex or age. We also found a trend of association of the 2-repeat allele
with PG. The discovery of a functional polymorphism in the dopamine D4 receptor gene (DRD4,
OMIM *126452) (Van Tol, et al., 1992) and its higher expression in the prefrontal cortex and temporo-
limbic regions (Mulcrone & Kerwin, 1997) made DRD4 one of the most investigated genes in behavior
and psychiatry. Several studies reported associations of this polymorphism with impulsive personality
traits (Becker, Laucht, El-Faddagh, & Schmidt, 2005; LaHoste, et al., 1996; Laucht, Becker, Blomeyer,
& Schmidt, 2007), addictions (Hill, Zezza, Wipprecht, Xu, & Neiswanger, 1999), and impulse control
disorders (Comings, et al., 1999; Perez de Castro, Ibanez, Torres, Saiz-Ruiz, & Fernandez-Piqueras,
1997) with conflicting results. Thus far, the most consistent findings were reported for attention-deficit
hyperactivity disorder (LaHoste, et al., 1996), as confirmed by meta-analyses (Bobb, Castellanos,
Addington, & Rapoport, 2005; D. Li, Sham, Owen, & He, 2006; Thapar, Langley, Owen, &
O'Donovan M, 2007).
In the previous decade, there was preliminary evidence of an inverse relationship between
length of repeats, clozapine biding affinity, and gene expression levels (Asghari, et al., 1995;
Jovanovic, Guan, & Van Tol, 1999; Schoots & Van Tol, 2003), and various studies compared the
presence of the 7-repeat allele against short allelic variants of this polymorphism. There is evidence
that transcripts from 7 and 2-repeat alleles have a lower potency when coupling with adenylyl-cyclase
compared with transcripts from 4 and 10-repeat alleles respectively (Asghari, et al., 1995; Jovanovic, et
al., 1999).
In vitro experiments suggest that the 2 and the 7 forms of the D4 receptor (see Figure 7)
present higher rates of degradation due to their structure rigidity and length, respectively (Van
Craenenbroeck, et al., 2005). These findings challenge the initial view that the length of the repeats is
inversely associated with gene function. Another factor that increases the complexity in the study of
DRD4 exon III VNTR is the fact that there is variation in the sequences within the alleles (Ding, et al.,
2002), i.e.
not all individuals with the 7-repeat allele will have the exact same sequence within the
allele. Thus, since our initial results indicate an association between PG severity and the 7-repeat allele,
and a marginal association between PG severity and the 2-repeat allele,
we hypothesize that
variations in the sequence of the 2- and 7-repeat alleles of DRD4 exon III VNTR polymorphism
will better predict PG severity.
2.Research Design and Methodological Approach:
2.1 Sample:
Subjects were assessed for PG diagnosis through the South Oaks Gambling Screen (SOGS) (Lesieur &
Blume, 1987) or the Problem Gambling Severity Index (PGSI) (Ferris & Wynne, 2001), resulting in a
sample of 541 PG subjects (highest lifetime PGSI or SOGS ≥3; with either positive or negative
screening for lifetime substance misuse) and 214 control subjects (highest lifetime PGSI or SOGS =0,
negative screening for lifetime substance misuse). Clinical data on this sample was obtained in our
previous OPGRC funded projects. All subjects were assessed through the Structured Clinical Interview
Diagnosis in Psychiatry based on DSM-IV criteria (SCID-NP). The SCID is a well tested, reliable and
widely used instrument in psychiatric research. It provides an assessment of lifetime diagnosis of major
psychiatric disorders (mood disorders, psychotic disorders, anxiety disorders and substance addiction).
The SCID is used worldwide (Amaral & Malbergiera, 2004; Chung, Tso, Cheung, & Wong, 2008;
Healey, Kneebone, Carroll, & Anderson, 2008; Hodgins, Dufour, & Armstrong, 2000; Nilsson &
Svedin, 2006; Rueda-Jaimes, et al., 2007; Torrens, Serrano, Astals, Perez-Dominguez, & Martin-
Santos, 2004; Whelan-Goodinson, Ponsford, & Schonberger, 2008) and is considered as the gold-
standard for diagnosis in psychiatric research, thus also being used to validate other diagnostic
instruments (Cassidy, Schmitz, & Malla, 2008; Healey, et al., 2008.; Sanchez-Villegas, et al., 2008).
Subjects were considered to present substance misuse if they answer positively to all three SCID/NP
screening questions for alcohol abuse and/or substance dependence. Noteworthy is the fact that tobacco
dependence was not investigated, as it was not part of SCID-NP.
2.2 Gene and Polymorphism (SNP) Selection:
The main KARG database pathways described above were the initial source for gene selection. Genes
in key positions in the pathways or genes that had been previously associated with PG in our studies
were selected. Each gene was then entered into the International Haplotype Map Project database
(www.hapmap.org) and available genotypic data for each gene was downloaded and analyzed for
detection of haplotypes.
Haplotype detection was performed through the software Haploview version 4.2 (Barrett, Fry, Maller,
& Daly, 2005). After haplotypes were detected within a gene, we proceeded to the selection of
tagSNPs. TagSNPs are SNPs (variants, polymorphisms) that are in high linkage disequilibrium (LD)
with other SNPs within a haplotype block and are chosen in order to reduce genotyping requirements
by eliminating redundancy in the information provided by SNPs in high LD. Haploview software
program finds the smallest set of tag SNPs that meets the requirements regarding minor allele
frequency (MAF), minimum value of r2 (linkage disequilibrium), and minimum distance between
SNPs. We selected tagSNPs with a minimum r2 of 0.8, MAF of 0.15 and a minimum distance between
SNPs of 60 base-pairs was required for accurate assay design.
After determining tagSNPs for all selected genes, we proceeded to test genetic assays for each tagSNP
in order to ensure that the assays would perform adequately. During this process, some of the selected
genes and tagSNPs had to be eliminated and substituted because it was not possible to design an assay.
Our final list included 40 genes and a total of 384 tagSNPs (Table 1) that were genotyped using an
Illumina® platform.
Figures 1-5 represent the five signalling pathways as described by Li et al. (2008). Below is a list of
genes that were selected for our study from each pathway. Please note that several of these genes are
present in more than one of the addiction-related pathways.
Genes selected from the Gap-junction pathway:
• dopamine receptor genes types 1 and 2 (DRD1, DRD2)
• metabotropic glutamate receptor genes 1 and 5 (GRM1, GRM5)
• serotonin receptor type 2 (HTR2A, HTR2C)
2 Linkage disequilibrium is a measure of nonrandom association between two or more alleles such that certain combinations of alleles are more likely to occur together on a chromosome than other combinations of alleles, i.e. the degree to which knowing the alleles of one SNP will accurately predict alleles of other SNPs. It is often parameterized as the squared correlation or r2 measure of linkage disequilibrium between two loci.
Genes selected from the MAPK pathway:
• mitogen-activated protein kinase kinase 1, 2, and 3 (MAP2K1, MAP2K2, MAP2K3)
• protein kinase, cAMP-dependent, catalytic – PKA family (PRKAC beta, alpha, and gamma)
• tumor necrosis factor receptor subfamily, member 1b – TNF family(TNFRSF1/ NBL1)
Genes selected from the GnRH pathway:
• gonadotropin-releasing hormone (GnRH1, GnRH2)
• phospholipase d, phosphatidylcholine-specific (PLD1, PLD2)
• protein kinase, cAMP-dependent, catalytic – PKA family (PRKAC beta, alpha, and gamma)
• insulin gene (INS)
Genes selected from Long-term potentiation pathway:
• calcium/calmodulin-dependent protein kinase (CAMK2A, CAMK2B, CAMK2D, CAMK2G),
• protein kinase, cAMP-dependent, catalytic – PKA family (PRKAC beta, alpha, and gamma),
• mitogen-activated protein kinase 1 (MAPK1 or ERK2).
Genes selected from Neuroactive-ligand pathway:
• dopamine receptor genes (DRD1, DRD2, DRD3, DRD4);
• metabotropic glutamate receptor genes (GRM1, GRM5),
• cannabinoid receptor gene (CNR1, CNR2),
• serotonin receptor genes (HTR1B, HTR2A, HTR3A, HTR3B, HTR6, HTR7).
Other genes that were selected based on our preliminary results:
• serotonin transporter gene (HTT/ SLC6A4),
• dopamine transporter gene (DAT/ SLC6A3),
• tyrosine hydroxylase gene (TH),
• tryptophan hydroxylase 2 gene (TPH2),
• cocaine- and amphetamine- regulated transcript (CARTPT)
• ankyrin repeat and kinase domain containing 1 gene (ANKK1) and tetratricopeptide repeat
domain 12 gene (TTC12). Due to their proximity DRD2, TTC12 and ANKK1 can be
considered as a cluster of genes.
2.3 Statistical Analysis:
Power calculations were performed through QUANTO power calculator (Gauderman & Morrison,
2006) and revealed that a case-control ratio of 1: 0.5, with 500 cases would have 80% power to detect
associations with an odds ratio of 1.5, considering a minor allele frequency of 0.15, and a population
prevalence of 0.04.
Statistical
significance was set at
α=0.001. Haplotype analysis was performed through Golden-Helix
SNP and Variation Suite version 7 (SVS v7). False Discovery Rate was applied for multiple-testing
The minimum requirements to include a tagSNP in the pool of markers to be analyzed were: genotype
calls >75%, HWE p ≥ 0.01, and haplotypes with a minimum 5% estimated haplotype frequency for
both cases and controls.
2.4 Sequencing of DRD4 exon III region:
Sequencing of the DRD4 exon III VNTR locus was performed at the Centre for Applied Genomics at
the Hospital for Sick Kids and in our laboratory using the ABI-Avant 3130 Genetic Analyzer for
Sequencing Analysis. All sequencing results were analyzed using SeqScape v.2.5. The results were
then reviewed for quality assurance and samples that presented dubious results were sent for re-
3. Results:
In our initial proposal, subjects could be considered as controls if their past year SOGS/PGSI scores
were = 0. However, several controls were re-assessed during out project and presented
lifetime
SOGS/PGSI scores of 1 to 2. Clinically, these subjects would be considered as low-risk gamblers and
had a lower likelihood of progressing to moderate and high-risk gambling categories. However, in
respect to their biological risk, it has been shown that genetic vulnerability for PG increases in
gamblers who have presented at least one of the DSM criteria for pathological gambling in comparison
to subjects who never met any DSM criteria for PG (Eisen, et al., 2001). Moreover, all controls that
presented a positive screening for alcohol abuse and/ or drug abuse (lifetime) were also excluded from
the analysis. Thus, our sample of control subjects was reduced to 214 subjects. Conversely, we were
able to identify 514 subjects that were considered as problem gamblers (minimum SOGS/ PGSI
lifetime scores ≥3). Amongst our cases, we also identified 99 PG subjects who also presented positive
screening for alcohol abuse and/ or drug abuse.
3.1 PG subjects (n=514) x Control Subjects (n=214)
For the comparison between our total sample of PG and control subjects, a total of 307 haplotypes
distributed along 40 genes were initially selected, based on our inclusion criteria of a minimum of 75%
genotype calls and MAF ≥ 0.15 (Table 1). Haplotype blocks for analysis were defined using the
confidence bounds method as described by Gabriel et al. (Gabriel, et al., 2002), in which 95%
confidence bounds on D' are generated and each comparison is called "strong LD", "inconclusive" or
"strong recombination". A block is created if 95% of informative (i.e. non-inconclusive) comparisons
are "strong LD". This definition allows for many overlapping blocks to be valid. The next step is to sort
the list of all possible blocks, start with the largest block and keep adding blocks as long as they don't
overlap with an already declared block. Only haplotype blocks with an estimated frequency >5% were
included in the analysis, so that the final selection comprised 303 tagSNPs distributed along 39 genes
(gene excluded: GnRH2).
Uncorrected p-values (≤0.001) show that haplotypes distributed across 13 genes were nominally
associated with PG (Table 2).
After FDR correction for multiple testing (α=0.001), two haplotypes on
CAMK2D, four haplotypes on
HTR2A, one haplotype on
PLD2, and one haplotype on
PRKACB
were significantly associated with PG.
FDR corrected p-values (between 0.002 and 0.01) revealed
association trends with DRD3 (dopamine receptor gene, subtype 3), GRM1 (metabotropic glutamate
receptor gene, subtype 1), HTR3A (serotonin receptor gene, subtype 3A), HTT/ SLC6A4 (serotonin
transporter gene), TTC12 (part of the ANKK1-DRD2-TTC12 cluster of genes), and CAMK2A
(calcium/calmodulin-dependent protein kinase, subtype 2A).
3.2 PG subjects with alcohol and/or drug abuse – PG-ADA (n=99) x Control Subjects (n=214)
For the comparison between PG subjects with positive screening for alcohol and/or substance abuse
(PG-ADA), a total of 298 tagSNPs across 39 genes (gene excluded: GnRH2) met our selection criteria
as described above.
3 D prime (D') is a scaled measure of the difference in frequency between observed number of haplotype pairs and the expected number. This measure is an estimator of linkage disequilibrium (LD).
Uncorrected p-values (≤0.001) show that 1 haplotype in:
CAMK2D is nominally associated with PG-
ADA (Table 3). The CAMK2D haplotype
rs2158196 _GCA is inversely associated with PG-ADA; i.e.
the haplotype might be protective, since its frequency is significantly (uncorrected p-value) lower in
PG-ADA subjects compared to healthy controls. None of these associations remained significant after
FDR correction for multiple testing.
3.3 PG subjects with alcohol and/or drug abuse – PG-ADA (n =99) x PG subjects without
substance abuse (n =257)
For the comparison between PG- ADA subjects and PG subjects without alcohol and/or drug abuse
history, a total of 298 tagSNPs across 39 genes (gene excluded: GnRH2) met our selection criteria as
described in item 3.1
Uncorrected p-values (≤0.001) show that
GRM1, CAMK2D, and DRD2 (one haplotype on each
gene) genes were nominally associated with the group of PG subjects without substance abuse history,
(Table 4). None of these associations remained significant after FDR correction for multiple testing.
As we first hypothesized, we found genetic associations with genes located on chromosomes 4
(CAMK2D), 5 (CAMK2A), and 17 (PLD2, HTR2A), which are chromosomes that have strong
evidence for harbouring susceptibility genes for addictions (M. D. Li & Burmeister, 2009), and on
chromosome 1 (PRKACB). We found nominal associations with genes located on chromosome 6
(GRM1), chromosome 11 (TTC12, DRD2), and chromosome 17 (HTT / SLC6A4).
Noteworthy is the
fact that across all group comparisons significant associations were found with CAMK2D,
which
is part of the pathway that interlinks the other 4 pathways.
3.4. DRD4 sequencing analysis
The DRD4 exon III VNTR locus was sequenced for 170 of our PG subjects.
We discovered a novel
single-nucleotide deletion in two out of eleven individuals homozygous for the 7-repeat allele in our
problem gambling sample (Figure 8). The novel single-nucleotide deletion variant was found in the
first repeat unit for one subject and in the third repeat unit for the second subject. In both cases the
deletion was of the "A" nucleotide. Although the deletions are located in two different repeat units the
deletions occur at the same position within the repeat units.
In silico analysis showed that this deletion would give rise to a shift in the reading frame, causing a
premature stop codon and truncated predicted amino acid sequence (Figure 9). This deletion was found
in two out of eleven subjects (18%) who had the 7-7 genotype. Both subjects were pathological
gamblers; however, due to the low frequency of the 7-7 genotype and of the deletion, we were not able
to verify whether the deletion was associated with PG severity. No deletions were found in any of the
subjects who had the 2-2 or 4-4 genotype.
4. Limitations and Changes to Original Proposal:
The main change to our original proposal is in regard to sample composition. As described in item 3
(page 14), in order to maintain a more strictly defined phenotype, the number of control subjects in our
sample was decreased (initially 400 controls, actual sample: 214), while we were able to increase the
number of cases (PG subjects – initially 400 subjects, actual sample: 514).
This change decreased our
power to detect associations with very small odds ratio (< 1.5). Nevertheless, we were still able to
detect associations that remained significant after correction for multiple testing.
We were granted a 6-month extension for our project. Our project was delayed initially due to
problems in importing genetic assays from the United States. Another important source of delay was
the legal process of authorizing DNA transfer between institutions, since part of our genotyping and
sequencing was done at another genetic laboratory in the University of Toronto. Finally, technical
difficulties with our sequencer delayed DRD4 sequency.
In regards to other limitations, we acknowledge that the sample size of our group of PG-ADA has
limited the interpretation of our results. At this point, we can say that the nominal associations found
(uncorrected p-values ≤ 0.001) can be regarded only as preliminary data. In this specific case,
preliminary data is important since this was the first study to investigate genetic associations with PG
subjects with positive screening for substance abuse.
The number of genes and tagSNPs selected could also be a source of concern. Our choice of genes
reflected the current knowledge on neuropathways involved in addictions
per se or in brain systems
that are closely related to PG and addictions. Each of these systems is composed of many parts, which
in turn are synthesized by a number of genes.
In fact, our choice of genes was parsimonious
compared to genome-wide association studies and reflects our concern with type I error. We
opted for a conservative α level (≤
0.001) and applied a multiple-testing correction that is
considered as conservative for candidate gene studies.
Despite limitations, this investigation presents many methodological advantages (sample size,
substantial rational for the choice of genes) compared to previously published PG genetic association
We are aware of the fact that different addictions (substance or behavioural) will not completely share
genetic vulnerability factors and that there is a significant environmental component in the
vulnerability for addiction disorders. We recognize that we investigated only a portion of the genetic
architecture of PG that overlaps with substance addiction, and that we selected only genes that are
considered as common vulnerability factors for addiction across different substances of abuse. It was
not our objective to investigate any environmental factors involved in PG.
As mentioned on page 16, the low frequency of the deletion found in subjects homozygous for the 7-7
genotype, prevented the investigation of a possible association of this deletion with PG. It was not
possible to know beforehand if we were going to find a new deletion and its frequency. Nevertheless,
the finding is valid and will be reported to the scientific community through publication on a peer-
reviewed scientific journal.
5. Discussion:
Is PG an addiction? If so, can a behavioural addiction affect the same brain systems as substances of
abuse? These questions have been the focus of attention of many researchers in the gambling field.
Recently, the Diagnostic and Statistical Manual for psychiatric disorders has acknowledged that
clinical and research data support the construct of PG as an addiction, thus incorporating PG under
addictive disorders in its next edition.
As outlined in section 1 (Introduction), imaging research has provided important evidence suggesting
that PG affects areas of the brains in a very similar fashion as addictive substances. However, to the
best of our knowledge, there has been no investigation on whether PG can induce changes in the brain
signalling pathways that have been traditionally regarded as induced by substances of abuse. In order to
provide initial data to answer this question, we have conducted a PG genetic association study on
whether genes on addiction-related signalling pathways were also associated with PG. For this project,
we were able to genotype 40 out of 396 genes involved in common addiction-related signalling
In summary, our results show that haplotypes on CAMK2D, PRKACB, HTR2A, and PLD2 were
significantly associated with PG when compared to control subjects in a sample of 514 PG subjects and
214 control subjects. Nominal associations (uncorrected p-values) were also found for other genes that
have been traditionally considered as the main candidate genes for PG: DRD3, GRM1, HTR3A, HTT/
SLC6A4, TTC12 (part of the ANKK1-DRD2-TTC12 cluster of genes).
In regards to our comparisons between PG-ADA and controls or PG subjects without substance abuse
history, we found also nominal associations with genes traditionally considered as the main candidate
genes for PG and addictions (DRD2, HTR2A, and GRM1), as well as with genes that had never been
investigated in regards to gambling behaviour: CAMK2A and CAMK2D.
Below we will provide an overview of the role of these genes in addictive processes and discuss the
implications of our findings in regards to advancing knowledge and to future research on the
neurobiology of PG. Finally, we will also discuss our findings regarding sequencing of the DRD4 exon
A. Calcium- and calmodulin- dependent protein kinase genes (CAMK2A and CAMK2D)
•
CAMK2A located on chromosome 5
•
CAMK2D located on chromosome 4
The CAMK2 family of genes is responsible for the production of calcium- and calmodulin-dependent
protein kinases. Protein Kinases act as regulators of cell function. Genes involved in protein kinases
translation (i.e., production) constitute one of the largest and most functionally diverse gene families.
Currently 518 human protein kinases have been indentified, with the vast majority of them belonging to
one superfamily. Each superfamily can be clustered into groups, families and sub-families, of
increasing sequence similarity and biochemical function. CAMK2 kinases (and thus CAMK2 genes)
are a subfamily of the CASK family, which is part of the larger CAMK group. By adding phosphate
groups to substrate proteins, they direct the activity, localization and overall function of many proteins,
and serve to orchestrate the activity of almost all cellular processes. Kinases are predominantly
important in signal transduction and co-ordination of complex cellular functions (Manning, Whyte,
Martinez, Hunter, & Sudarsanam, 2002). CAMK2 is involved in several aspects of neuronal function,
including neuroplasticity, gene expression, and neurotransmitter synthesis and release (Lee & Messing,
CAMK2A and CAMK2D are part of the long-term potentiation and GnRH signalling pathways
(Figures 2 and 3, respectively). Figure 2 shows that CAMK2 genes directly regulate ionotropic
glutamate receptor (AMPAR/ GRIN) cell cycle, thus affecting the Neuroactive-ligand receptor
interaction pathway, i.e. CAMK2 modulation affects how AMPAR will respond to glutamate. CAMK2
also modulates D1 receptor cell cycle (not represented in Figure 2).
Figure 3 illustrates how CAMK2 genes ultimately regulate gene expression and secretion of
gonadotropins, thus directly affecting GnRHR1 and 2 genes on the Neuroactive-ligand receptor
interaction pathway.
Ultimately, the 5 common pathways for addiction are integrated with each other
through fast and slow positive feedbacks loops that are all interlinked through CAMK2 (C. Y. Li, et
CAMK2 has been reported to be involved in several addictive processes: sensitization, drug tolerance
and self-administration, withdrawal and relapse (Lee & Messing, 2008).
B. Serotonin receptor 2A gene (HTR2A):
•
HTR2A located on chromosome 13
Serotonin (5-HT) activity is associated with several behaviours such as behavioral inhibition (Coccaro,
et al., 1989; Stein, Hollander, & Liebowitz, 1993), sensory reactivity (Sheard & Aghajanian, 1968) ,
sleep, sexual behaviour, and cognitive function (Patterson, et al., 1996; Ressler & Nemeroff, 2000). At
least 14 subtypes of 5-HT receptors have been cloned and identified. The excitatory 5- HT2 receptor
class is predominantly found on postsynaptic neurons, and activates phospholipase C. As can be seen
on figure 5, phospholipase C (PLC) activates protein kinase C (PKC), also involved in addictive
processes (see item C below).
Serotonin does not directly participate in motivation-reward, but exerts influence through its effects on
the dopamine system. Application of 5-HT onto dopaminergic neurons from the VTA (part of the
brain's reward system) increased their firing rate in vitro, an effect that was attributed to action of 5-HT
on HTR2 receptors (Pessia, Jiang, North, & Johnson, 1994). As shown in figures 1-5, serotonin
receptors are part of the neuroligand-receptor interaction (figure 1) and gap junction pathways (figure
5). Even though it has become common knowledge that 5-HT receptors are involved in behavioural
inhibition and cognitive functions, previous candidate gene studies had focused on the investigation of
the serotonin transporter (HTT). This is the first candidate gene study in PG to investigate and report an
association with serotonin receptor gene 2A (HTR2A).
C. Phospholipase D2 gene (PLD2):
•
PLD2 located on chromosome 17
There are two types of phospholipase genes: PLD1 and PLD2. Both genes synthesize pospholipase D,
an enzyme that produces phosphatidic acid (PA) through hydrolyzation processes. PA is further
metabolized into diacylglycerol (DAG), which regulates certain types of protein kinase C (PKC). Thus,
PLD genes regulate PKC activity through the production of PA and DAG. Activation of PKC by
calcium ions and the second messenger diacylglycerol is thought to
play a central role in the induction
of cellular responses to a variety of ligand-receptor systems (glutamate ionotropic receptors,
dopamine D2 receptor, canabinoid receptor type 1, and nicotinic cholinergic receptors) and in the
regulation of cellular responsiveness to external stimuli.
The prefrontal cortex (PFC) is a brain region that regulates thought, behavior, and emotion using
representational knowledge, operations often referred to as working memory. Birnbaum et al.
(Birnbaum, et al., 2004) have tested the influence of PKC on intracellular signaling on PFC cognitive
function and showed that high levels of PKC activity in prefrontal cortex (for instance, induced by
stress) result in significant impairment of measures of working memory. These data suggest that
excessive PKC activation can disrupt PFC regulation of behavior and thought, possibly contributing
to signs of distractibility, impaired judgment, impulsivity, and thought disorder. Distractibility,
impaired judgment, and impulsivity are well recognized features of PG (Cavedini, Riboldi, Keller,
D'Annucci, & Bellodi, 2002; Fuentes, Tavares, Artes, & Gorenstein, 2006; Goudriaan, Oosterlaan, de
Beurs, & van den Brink, 2005; Petry, 2001), and
this finding raises the possibility that working
memory deficits in PG and substance addiction occur through the same pathway (PLD inducing
PKC activation; PKC regulation cellular response to dopamine, glutamate receptors).
Similarly to CAMK2, PKC has also been involved in sensitization, drug tolerance, and drug self-
administration and withdrawal (Lee & Messing, 2008).
D. Protein kinase, cAMP-dependent, catalytic, beta gene (PRKACB)_
•
PRKACB located on chromosome 1
The PRKACB gene synthesizes the β-catalytic isoform of the c-AMP dependent protein kinase A
(PKA). PKA is inactive in its natural state, and is activated when c-AMP (produced by adenylyl
cyclase) binds to its regulatory region (Lee & Messing, 2008).
It has been shown that addictive
substances promote an acute increase in extracellular levels of dopamine in the nucleus accumbens,
which stimulates adenylyl cyclase (producer of c-AMP) and PKA via D1 receptors (dopamine
receptor type 1).
Substances that act as agonists in
the D1 receptor together with PKA activation, increase the
cell
surface expression of glutamate receptor 1 (ionotropic glutamate receptor 1, AMPA 1) in the nucleus
accumbens and hippocampus of rats (Gao, Sun, & Wolf, 2006). Increasing the expression of the
glutamate receptor increases synaptic strength. Thus,
PKA activation may provide a mechanism for
substance-induced neuroplasticity. Similarly to CAMK2 and PKC, animal models have demonstrated
PKA's involvement in sensitization, drug tolerance, and drug self-administration and withdrawal (Lee
& Messing, 2008).
E. DRD4 exon III VNTR sequencing:
Our sequencing efforts on DRD4 have revealed a deletion in the 7-repeat allele of DRD4 exon III
VNTR. Moreover, our results suggest that this deletion can interrupt the synthesis of the receptor,
which could result in a "defective" D4 receptors (on 7-7 allele carriers) or in a decreased production of
D4 receptors.
This is the first time that a deletion that would alter gene transcription has been
described for the 7-repeat allele of DRD4 exon III VNTR.
The relatively low frequency of the 7-7 genotype in the general population (3%) highlights the need for
heterozygotes to be sequenced. As of yet, PG subjects who are heterozygous for the 7-repeat allele (2-
7, 4-7) were not sequenced due to cost limitations. Our future plans include sequencing 7-repeat
heterozygotes and cloning sequences that present this deletion. After cloning,
in vitro functional studies
would provide experimental evidence of the effect of this deletion on the D4 receptor.
In conclusion, this is the largest genetic association study conducted on PG until now, and the first to
investigate the association between PG and genes involved in addiction signalling pathways. Our
original proposal indicated two major applications for this investigation: 1. to provide better
understanding of the neurobiology of PG, and 2. to provide information in regards to processes that are
amenable to pharmacological intervention. As discussed above, our results provide further insight into
the neurobiology of PG. By taking a systems approach in selecting candidate genes we were able to
identify new genes that had not been previously investigated in PG and that provide clues regarding
shared genetic pathways between PG and substance addictions.
As discussed above, CAMK2A,
PRKACB, HTR2A, and PLD2 genes have important roles in synthesizing and or regulating proteins,
enzymes, and second messengers that interact with each other and induce addiction-related
neurochemical changes. These genetic findings will likely inform future neurobiological investigation
into shared protein and enzymatic pathways between PG and substance addictions.
It is important to acknowledge that our results do not invalidate previous genetic associations with PG
and substance addiction, since our sample had the power to detect genes with higher effect sizes.
Genes, for which nominal associations were found, especially in the comparisons with PG-ADA,
deserve further investigation in larger samples where comorbidity with substance use disorders has
been documented.
Our findings can also help interpret results from previous genetic association studies in PG. For
instance, our group had previously reported an association of PG with the DRD1 gene in a family
sample (da Silva Lobo, et al., 2007), and trends for association on the DRD2/ ANKK1
TaqIA/rs1800497 polymorphism (p=0.01) and the haplotype flanking DRD2 (G/C/A
rs11604671/rs4938015/rs2303380) (Lobo, et al., 2010). Other studies had reported also associations
with DRD2/ ANKK1 TaqIA in smaller samples of PG (Comings, et al., 1997; Comings, et al., 1996; da
Silva Lobo, et al., 2007). Our results show that genes that regulate or are regulated by DRD1 and
DRD2 are significantly associated with PG. This suggests that, although previously reported
associations with DRD1 and DRD2 are valid, genes involved in up-stream and down-stream dopamine
signalling have a greater effect (have a stronger association) on PG. The same can be said in regards to
other neurotransmitter receptor genes such as glutamate receptor genes.
Also, and perhaps more importantly, our results have provided information in regards to processes that
are amenable to pharmacological intervention.
The association of PG with CAMK2D and PRKACB
and PLD2 (directly or indirectly involved in protein kinases synthesis) indicates that protein-kinase
inhibitors could potentially be used in the treatment of PG. The recent availability of an animal model
for gambling behaviour allows pre-clinical testing of protein-kinase inhibitors that are currently under
development, which would be the appropriate next step in the investigation of our findings.
6. References:
Abrieu, A., Doree, M., & Fisher, D. (2001). The interplay between cyclin-B-Cdc2 kinase (MPF) and
MAP kinase during maturation of oocytes.
J Cell Sci, 114(Pt 2), 257-267.
Amaral, R. A., & Malbergiera, A. (2004). [Evaluation of a screening test for alcohol-related problems
(CAGE) among employees of the Campus of the University of Sao Paulo].
Rev Bras Psiquiatr, 26(3), 156-163.
4 The polymorphism formerly known as DRD2 TaqIA is actually located in the ANKK1 gene, neighbouring DRD2.
Asghari, V., Sanyal, S., Buchwaldt, S., Paterson, A., Jovanovic, V., & Van Tol, H. H. (1995).
Modulation of intracellular cyclic AMP levels by different human dopamine D4 receptor variants.
J Neurochem, 65(3), 1157-1165.
Barrett, J. C., Fry, B., Maller, J., & Daly, M. J. (2005). Haploview: analysis and visualization of LD
and haplotype maps.
Bioinformatics, 21(2), 263-265.
Becker, K., Laucht, M., El-Faddagh, M., & Schmidt, M. H. (2005). The dopamine D4 receptor gene
exon III polymorphism is associated with novelty seeking in 15-year-old males from a high-risk community sample.
J Neural Transm, 112(6), 847-858.
Birnbaum, S. G., Yuan, P. X., Wang, M., Vijayraghavan, S., Bloom, A. K., Davis, D. J., et al. (2004).
Protein kinase C overactivity impairs prefrontal cortical regulation of working memory.
Science, 306(5697), 882-884.
Black, D. W., & Moyer, T. (1998). Clinical features and psychiatric comorbidity of subjects with
pathological gambling behavior.
Psychiatr Serv, 49(11), 1434-1439.
Blanco, C., Moreyra, P., Nunes, E. V., Saiz-Ruiz, J., & Ibanez, A. (2001). Pathological gambling:
addiction or compulsion?
Semin Clin Neuropsychiatry, 6(3), 167-176.
Bland, R. C., Newman, S. C., Orn, H., & Stebelsky, G. (1993). Epidemiology of pathological gambling
in Edmonton.
Can J Psychiatry, 38(2), 108-112.
Bobb, A. J., Castellanos, F. X., Addington, A. M., & Rapoport, J. L. (2005). Molecular genetic studies
of ADHD: 1991 to 2004.
Am J Med Genet B Neuropsychiatr Genet, 132(1), 109-125.
Brandman, O., Ferrell, J. E., Jr., Li, R., & Meyer, T. (2005). Interlinked fast and slow positive feedback
loops drive reliable cell decisions.
Science, 310(5747), 496-498.
Cardinal, R. N., & Everitt, B. J. (2004). Neural and psychological mechanisms underlying appetitive
learning: links to drug addiction.
Curr Opin Neurobiol, 14(2), 156-162.
Cassidy, C. M., Schmitz, N., & Malla, A. (2008). Validation of the alcohol use disorders identification
test and the drug abuse screening test in first episode psychosis.
Can J Psychiatry, 53(1), 26-33.
Cavedini, P., Riboldi, G., Keller, R., D'Annucci, A., & Bellodi, L. (2002). Frontal lobe dysfunction in
pathological gambling patients.
Biol Psychiatry, 51(4), 334-341.
Chambers, R. A., & Potenza, M. N. (2003). Neurodevelopment, impulsivity, and adolescent gambling.
J Gambl Stud, 19(1), 53-84.
Chambers, R. A., Taylor, J. R., & Potenza, M. N. (2003). Developmental neurocircuitry of motivation
in adolescence: a critical period of addiction vulnerability.
Am J Psychiatry, 160(6), 1041-1052.
Chung, K. F., Tso, K. C., Cheung, E., & Wong, M. (2008). Validation of the Chinese version of the
Mood Disorder Questionnaire in a psychiatric population in Hong Kong.
Psychiatry Clin Neurosci, 62(4), 464-471.
Coccaro, E. F., Siever, L. J., Klar, H. M., Maurer, G., Cochrane, K., Cooper, T. B., et al. (1989).
Serotonergic studies in patients with affective and personality disorders. Correlates with suicidal and impulsive aggressive behavior.
Arch Gen Psychiatry, 46(7), 587-599.
Comings, D. E., Gade, R., Wu, S., Chiu, C., Dietz, G., Muhleman, D., et al. (1997). Studies of the
potential role of the dopamine D1 receptor gene in addictive behaviors.
Mol Psychiatry, 2(1), 44-56.
Comings, D. E., Gonzalez, N., Wu, S., Gade, R., Muhleman, D., Saucier, G., et al. (1999). Studies of
the 48 bp repeat polymorphism of the DRD4 gene in impulsive, compulsive, addictive behaviors: Tourette syndrome, ADHD, pathological gambling, and substance abuse.
Am J Med Genet, 88(4), 358-368.
Comings, D. E., Rosenthal, R. J., Lesieur, H. R., Rugle, L. J., Muhleman, D., Chiu, C., et al. (1996). A
study of the dopamine D2 receptor gene in pathological gambling.
Pharmacogenetics, 6(3), 223-234.
Crockford, D. N., & el-Guebaly, N. (1998). Psychiatric comorbidity in pathological gambling: a critical
review.
Can J Psychiatry, 43(1), 43-50.
Crockford, D. N., Goodyear, B., Edwards, J., Quickfall, J., & el-Guebaly, N. (2005). Cue-induced brain
activity in pathological gamblers.
Biol Psychiatry, 58(10), 787-795.
Cunningham-Williams, R. M., Cottler, L. B., Compton, W. M., 3rd, & Spitznagel, E. L. (1998). Taking
chances: problem gamblers and mental health disorders--results from the St. Louis Epidemiologic Catchment Area Study.
Am J Public Health, 88(7), 1093-1096.
da Silva Lobo, D. S., Vallada, H. P., Knight, J., Martins, S. S., Tavares, H., Gentil, V., et al. (2007).
Dopamine genes and pathological gambling in discordant sib-pairs.
J Gambl Stud, 23(4), 421-433.
Daghestani, A. N., Elenz, E., & Crayton, J. W. (1996). Pathological gambling in hospitalized substance
abusing veterans.
J Clin Psychiatry, 57(8), 360-363.
de Ruiter, M. B., Veltman, D. J., Goudriaan, A. E., Oosterlaan, J., Sjoerds, Z., & van den Brink, W.
(2009). Response perseveration and ventral prefrontal sensitivity to reward and punishment in male problem gamblers and smokers.
Neuropsychopharmacology, 34(4), 1027-1038.
Ding, Y. C., Chi, H. C., Grady, D. L., Morishima, A., Kidd, J. R., Kidd, K. K., et al. (2002). Evidence
of positive selection acting at the human dopamine receptor D4 gene locus.
Proc Natl Acad Sci U S A, 99(1), 309-314.
Eisen, S. A., Lin, N., Lyons, M. J., Scherrer, J. F., Griffith, K., True, W. R., et al. (1998). Familial
influences on gambling behavior: an analysis of 3359 twin pairs.
Addiction, 93(9), 1375-1384.
Eisen, S. A., Slutske, W. S., Lyons, M. J., Lassman, J., Xian, H., Toomey, R., et al. (2001). The
genetics of pathological gambling.
Semin Clin Neuropsychiatry, 6(3), 195-204.
Feigelman, W., Kleinman, P. H., Lesieur, H. R., Millman, R. B., & Lesser, M. L. (1995). Pathological
gambling among methadone patients.
Drug Alcohol Depend, 39(2), 75-81.
Feigelman, W., Wallisch, L. S., & Lesieur, H. R. (1998). Problem gamblers, problem substance users,
and dual-problem individuals: an epidemiological study.
Am J Public Health, 88(3), 467-470.
Ferris , J., & Wynne, H. (2001). The Canadian Problem Gambling Index: Final report.
Canadian
Centre on Substance Abuse.
Fuentes, D., Tavares, H., Artes, R., & Gorenstein, C. (2006). Self-reported and neuropsychological
measures of impulsivity in pathological gambling.
J Int Neuropsychol Soc, 12(6), 907-912.
Gabriel, S. B., Schaffner, S. F., Nguyen, H., Moore, J. M., Roy, J., Blumenstiel, B., et al. (2002). The
structure of haplotype blocks in the human genome.
Science, 296(5576), 2225-2229.
Gao, C., Sun, X., & Wolf, M. E. (2006). Activation of D1 dopamine receptors increases surface
expression of AMPA receptors and facilitates their synaptic incorporation in cultured hippocampal neurons.
J Neurochem, 98(5), 1664-1677.
Gauderman, W., & Morrison, J. (2006). QUANTO 1.1: A computer program for power and sample size
calculations for genetic-epidemiology studies.
Goudriaan, A. E., Oosterlaan, J., de Beurs, E., & van den Brink, W. (2005). Decision making in
pathological gambling: a comparison between pathological gamblers, alcohol dependents, persons with Tourette syndrome, and normal controls.
Brain Res Cogn Brain Res, 23(1), 137-151.
Grant, J. E., Kim, S. W., & Hartman, B. K. (2008). A Double-Blind, Placebo-Controlled Study of the
Opiate Antagonist Naltrexone in the Treatment of Pathological Gambling Urges.
J Clin Psychiatry, e1-e7.
Grant, J. E., Potenza, M. N., Hollander, E., Cunningham-Williams, R., Nurminen, T., Smits, G., et al.
(2006). Multicenter investigation of the opioid antagonist nalmefene in the treatment of pathological gambling.
Am J Psychiatry, 163(2), 303-312.
Hall, G. W., Carriero, N. J., Takushi, R. Y., Montoya, I. D., Preston, K. L., & Gorelick, D. A. (2000).
Pathological gambling among cocaine-dependent outpatients.
Am J Psychiatry, 157(7), 1127-1133.
Healey, A. K., Kneebone, II, Carroll, M., & Anderson, S. J. (2008). A preliminary investigation of the
reliability and validity of the Brief Assessment Schedule Depression Cards and the Beck Depression Inventory-Fast Screen to screen for depression in older stroke survivors.
Int J Geriatr Psychiatry, 23(5), 531-536.
Herzog, D. B., Keller, M. B., Lavori, P. W., Kenny, G. M., & Sacks, N. R. (1992). The prevalence of
personality disorders in 210 women with eating disorders.
J Clin Psychiatry, 53(5), 147-152.
Hill, S. Y., Zezza, N., Wipprecht, G., Xu, J., & Neiswanger, K. (1999). Linkage studies of D2 and D4
receptor genes and alcoholism.
Am J Med Genet, 88(6), 676-685.
Hodgins, D. C., Dufour, M., & Armstrong, S. (2000). The reliability and validity of the inventory to
diagnose depression in alcohol-dependent men and women.
J Subst Abuse, 11(4), 369-378.
Hollander, E., Pallanti, S., Baldini Rossi, N., Sood, E., Baker, B. R., & Buchsbaum, M. S. (2005).
Imaging monetary reward in pathological gamblers.
World J Biol Psychiatry, 6(2), 113-120.
Jacobs, D. F. (1989). Children of problem gamblers.
Journal of Gambling Behavior, 5, 261-268. Jones, S., & Bonci, A. (2005). Synaptic plasticity and drug addiction.
Curr Opin Pharmacol, 5(1), 20-
Jovanovic, V., Guan, H. C., & Van Tol, H. H. (1999). Comparative pharmacological and functional
analysis of the human dopamine D4.2 and D4.10 receptor variants.
Pharmacogenetics, 9(5), 561-568.
Kalivas, P. W., & Volkow, N. D. (2005). The neural basis of addiction: a pathology of motivation and
choice.
Am J Psychiatry, 162(8), 1403-1413.
Karkowski, L. M., Prescott, C. A., & Kendler, K. S. (2000). Multivariate assessment of factors
influencing illicit substance use in twins from female-female pairs.
Am J Med Genet, 96(5), 665-670.
Kausch, O. (2003). Patterns of substance abuse among treatment-seeking pathological gamblers.
J
Subst Abuse Treat, 25(4), 263-270.
Kelley, A. E. (1999). Functional specificity of ventral striatal compartments in appetitive behaviors.
Ann N Y Acad Sci, 877, 71-90.
Kendler, K. S., Jacobson, K. C., Prescott, C. A., & Neale, M. C. (2003). Specificity of genetic and
environmental risk factors for use and abuse/dependence of cannabis, cocaine, hallucinogens, sedatives, stimulants, and opiates in male twins.
Am J Psychiatry, 160(4), 687-695.
Kim, S. W., Grant, J. E., Adson, D. E., & Shin, Y. C. (2001). Double-blind naltrexone and placebo
comparison study in the treatment of pathological gambling.
Biol Psychiatry, 49(11), 914-921.
LaHoste, G. J., Swanson, J. M., Wigal, S. B., Glabe, C., Wigal, T., King, N., et al. (1996). Dopamine
D4 receptor gene polymorphism is associated with attention deficit hyperactivity disorder.
Mol Psychiatry, 1(2), 121-124.
Laucht, M., Becker, K., Blomeyer, D., & Schmidt, M. H. (2007). Novelty seeking involved in
mediating the association between the dopamine D4 receptor gene exon III polymorphism and heavy drinking in male adolescents: results from a high-risk community sample.
Biol Psychiatry, 61(1), 87-92.
Ledgerwood, D. M., & Downey, K. K. (2002). Relationship between problem gambling and substance
use in a methadone maintenance population.
Addict Behav, 27(4), 483-491.
Lee, A. M., & Messing, R. O. (2008). Protein kinases and addiction.
Ann N Y Acad Sci, 1141, 22-57. Lesieur, H. R., & Blume, S. B. (1987). The South Oaks Gambling Screen (SOGS): a new instrument
for the identification of pathological gamblers.
Am J Psychiatry, 144(9), 1184-1188.
Lesieur, H. R., Blume, S. B., & Zoppa, R. M. (1986). Alcoholism, drug abuse, and gambling.
Alcohol
Clin Exp Res, 10(1), 33-38.
Lesieur, H. R., & Heineman, M. (1988). Pathological gambling among youthful multiple substance
abusers in a therapeutic community.
Br J Addict, 83(7), 765-771.
Li, C. Y., Mao, X., & Wei, L. (2008). Genes and (common) pathways underlying drug addiction.
PLoS
Comput Biol, 4(1), e2.
Li, D., Sham, P. C., Owen, M. J., & He, L. (2006). Meta-analysis shows significant association
between dopamine system genes and attention deficit hyperactivity disorder (ADHD).
Hum Mol Genet, 15(14), 2276-2284.
Li, M. D., & Burmeister, M. (2009). New insights into the genetics of addiction.
Nat Rev Genet, 10(4),
Lobo, D. S., Souza, R. P., Tong, R. P., Casey, D. M., Hodgins, D. C., Smith, G. J., et al. (2010).
Association of functional variants in the dopamine D2-like receptors with risk for gambling behaviour in healthy Caucasian subjects.
Biol Psychol, 85(1), 33-37.
Lynch, W. J., Maciejewski, P. K., & Potenza, M. N. (2004). Psychiatric correlates of gambling in
adolescents and young adults grouped by age at gambling onset.
Arch Gen Psychiatry, 61(11), 1116-1122.
Maccallum, F., & Blaszczynski, A. (2002). Pathological gambling and comorbid substance use.
Aust N
Z J Psychiatry, 36(3), 411-415.
Manning, G., Whyte, D. B., Martinez, R., Hunter, T., & Sudarsanam, S. (2002). The protein kinase
complement of the human genome.
Science, 298(5600), 1912-1934.
Marks, I. (1990). Behavioural (non-chemical) addictions.
Br J Addict, 85(11), 1389-1394. Mason, B. J., Salvato, F. R., Williams, L. D., Ritvo, E. C., & Cutler, R. B. (1999). A double-blind,
placebo-controlled study of oral nalmefene for alcohol dependence.
Arch Gen Psychiatry, 56(8), 719-724.
McCormick, R. A., Russo, A. M., Ramirez, L. F., & Taber, J. I. (1984). Affective disorders among
pathological gamblers seeking treatment.
Am J Psychiatry, 141(2), 215-218.
Miller, S., Yasuda, M., Coats, J. K., Jones, Y., Martone, M. E., & Mayford, M. (2002). Disruption of
dendritic translation of CaMKIIalpha impairs stabilization of synaptic plasticity and memory consolidation.
Neuron, 36(3), 507-519.
Mulcrone, J., & Kerwin, R. W. (1997). The regional pattern of D4 gene expression in human brain.
Neurosci Lett, 234(2-3), 147-150.
Nilsson, D., & Svedin, C. G. (2006). Evaluation of the Swedish version of Dissociation Questionnaire
(DIS-Q), Dis-Q-Sweden, among adolescents.
J Trauma Dissociation, 7(3), 65-89.
Noda, Y., & Nabeshima, T. (2004). Opiate physical dependence and N-methyl-D-aspartate receptors.
Eur J Pharmacol, 500(1-3), 121-128.
Patterson, S. L., Abel, T., Deuel, T. A., Martin, K. C., Rose, J. C., & Kandel, E. R. (1996).
Recombinant BDNF rescues deficits in basal synaptic transmission and hippocampal LTP in BDNF knockout mice.
Neuron, 16(6), 1137-1145.
Pelchat, M. L. (2002). Of human bondage: food craving, obsession, compulsion, and addiction.
Physiol
Behav, 76(3), 347-352.
Perez de Castro, I., Ibanez, A., Torres, P., Saiz-Ruiz, J., & Fernandez-Piqueras, J. (1997). Genetic
association study between pathological gambling and a functional DNA polymorphism at the D4 receptor gene.
Pharmacogenetics, 7(5), 345-348.
Pessia, M., Jiang, Z. G., North, R. A., & Johnson, S. W. (1994). Actions of 5-hydroxytryptamine on
ventral tegmental area neurons of the rat in vitro.
Brain Res, 654(2), 324-330.
Petry, N. M. (2001). Substance abuse, pathological gambling, and impulsiveness.
Drug Alcohol
Depend, 63(1), 29-38.
Petry, N. M., Stinson, F. S., & Grant, B. F. (2005). Comorbidity of DSM-IV pathological gambling and
other psychiatric disorders: results from the National Epidemiologic Survey on Alcohol and Related Conditions.
J Clin Psychiatry, 66(5), 564-574.
Potenza, M. N. (2001). The neurobiology of pathological gambling.
Semin Clin Neuropsychiatry, 6(3),
Potenza, M. N., Steinberg, M. A., Skudlarski, P., Fulbright, R. K., Lacadie, C. M., Wilber, M. K., et al.
(2003). Gambling urges in pathological gambling: a functional magnetic resonance imaging study.
Arch Gen Psychiatry, 60(8), 828-836.
Ramirez, L., McCormick, R., Russo, A., & Taber, J. (1984). Patterns of substance abuse in pathological
gamblers seeking treatment.
Addict Behav, 8, 425-428.
Ressler, K. J., & Nemeroff, C. B. (2000). Role of serotonergic and noradrenergic systems in the
pathophysiology of depression and anxiety disorders.
Depress Anxiety, 12 Suppl 1, 2-19.
Ross, H. E., Glaser, F. B., & Germanson, T. (1988). The prevalence of psychiatric disorders in patients
with alcohol and other drug problems.
Arch Gen Psychiatry, 45(11), 1023-1031.
Roy, A., Adinoff, B., Roehrich, L., Lamparski, D., Custer, R., Lorenz, V., et al. (1988). Pathological
gambling. A psychobiological study.
Arch Gen Psychiatry, 45(4), 369-373.
Rueda-Jaimes, G. E., Diaz-Martinez, L. A., Escobar-Sanchez, M., Franco-Lopez, J. A., Navarro-
Mancilla, A. A., & Cadena-Afanador, L. P. (2007). [Validation of the short version of the Leyton obsessional inventory for children and adolescents in Bucaramanga, Colombia].
Aten Primaria, 39(2), 75-80.
Sanchez-Villegas, A., Schlatter, J., Ortuno, F., Lahortiga, F., Pla, J., Benito, S., et al. (2008). Validity
of a self-reported diagnosis of depression among participants in a cohort study using the Structured Clinical Interview for DSM-IV (SCID-I).
BMC Psychiatry, 8, 43.
Schoots, O., & Van Tol, H. H. (2003). The human dopamine D4 receptor repeat sequences modulate
expression.
Pharmacogenomics J, 3(6), 343-348.
Shaffer, H. J., & Korn, D. A. (2002). Gambling and related mental disorders: a public health analysis.
Annu Rev Public Health, 23, 171-212.
Shaffer, H. J., LaPlante, D. A., LaBrie, R. A., Kidman, R. C., Donato, A. N., & Stanton, M. V. (2004).
Toward a syndrome model of addiction: multiple expressions, common etiology.
Harv Rev Psychiatry, 12(6), 367-374.
Sheard, M. H., & Aghajanian, G. K. (1968). Stimulation of midbrain raphe neurons: behavioral effects
of serotonin release.
Life Sci, 7(1), 19-25.
Slutske, W. S., Eisen, S., True, W. R., Lyons, M. J., Goldberg, J., & Tsuang, M. (2000). Common
genetic vulnerability for pathological gambling and alcohol dependence in men.
Arch Gen Psychiatry, 57(7), 666-673.
Slutske, W. S., Zhu, G., Meier, M. H., & Martin, N. G. (2010). Genetic and environmental influences
on disordered gambling in men and women.
Arch Gen Psychiatry, 67(6), 624-630.
Spunt, B. (2002). Pathological gambling and substance misuse.
Subst Use Misuse, 37(8-10), 1299-
Spunt, B., Lesieur, H., Hunt, D., & Cahill, L. (1995). Gambling among methadone patients.
Int J
Addict, 30(8), 929-962.
Stein, D. J., Hollander, E., & Liebowitz, M. R. (1993). Neurobiology of impulsivity and the impulse
control disorders.
J Neuropsychiatry Clin Neurosci, 5(1), 9-17.
Tang, L., Shukla, P. K., Wang, L. X., & Wang, Z. J. (2006). Reversal of morphine antinociceptive
tolerance and dependence by the acute supraspinal inhibition of Ca(2+)/calmodulin-dependent protein kinase II.
J Pharmacol Exp Ther, 317(2), 901-909.
Thapar, A., Langley, K., Owen, M. J., & O'Donovan M, C. (2007). Advances in genetic findings on
attention deficit hyperactivity disorder.
Psychol Med, 1-12.
Toneatto, T., & Brennan, J. (2002). Pathological gambling in treatment-seeking substance abusers.
Addict Behav, 27(3), 465-469.
Torrens, M., Serrano, D., Astals, M., Perez-Dominguez, G., & Martin-Santos, R. (2004). Diagnosing
comorbid psychiatric disorders in substance abusers: validity of the Spanish versions of the Psychiatric Research Interview for Substance and Mental Disorders and the Structured Clinical Interview for DSM-IV.
Am J Psychiatry, 161(7), 1231-1237.
Tsuang, M. T., Lyons, M. J., Meyer, J. M., Doyle, T., Eisen, S. A., Goldberg, J., et al. (1998). Co-
occurrence of abuse of different drugs in men: the role of drug-specific and shared vulnerabilities.
Arch Gen Psychiatry, 55(11), 967-972.
Valjent, E., Corbille, A. G., Bertran-Gonzalez, J., Herve, D., & Girault, J. A. (2006). Inhibition of ERK
pathway or protein synthesis during reexposure to drugs of abuse erases previously learned place preference.
Proc Natl Acad Sci U S A, 103(8), 2932-2937.
Van Craenenbroeck, K., Clark, S. D., Cox, M. J., Oak, J. N., Liu, F., & Van Tol, H. H. (2005). Folding
efficiency is rate-limiting in dopamine D4 receptor biogenesis.
J Biol Chem, 280(19), 19350-19357.
Van Tol, H. H., Wu, C. M., Guan, H. C., Ohara, K., Bunzow, J. R., Civelli, O., et al. (1992). Multiple
dopamine D4 receptor variants in the human population.
Nature, 358(6382), 149-152.
Vitaro, F., Brendgen, M., Ladouceur, R., & Tremblay, R. E. (2001). Gambling, delinquency, and drug
use during adolescence: mutual influences and common risk factors.
J Gambl Stud, 17(3), 171-190.
Volpicelli, J. R., Alterman, A. I., Hayashida, M., & O'Brien, C. P. (1992). Naltrexone in the treatment
of alcohol dependence.
Arch Gen Psychiatry, 49(11), 876-880.
Wang, J. Q., Fibuch, E. E., & Mao, L. (2007). Regulation of mitogen-activated protein kinases by
glutamate receptors.
J Neurochem, 100(1), 1-11.
Weinstock, J., Blanco, C., & Petry, N. M. (2006). Health correlates of pathological gambling in a
methadone maintenance clinic.
Exp Clin Psychopharmacol, 14(1), 87-93.
Welte, J., Barnes, G., Wieczorek, W., Tidwell, M. C., & Parker, J. (2001). Alcohol and gambling
pathology among U.S. adults: prevalence, demographic patterns and comorbidity.
J Stud Alcohol, 62(5), 706-712.
Whelan-Goodinson, R., Ponsford, J., & Schonberger, M. (2008). Validity of the Hospital Anxiety and
Depression Scale to assess depression and anxiety following traumatic brain injury as compared with the Structured Clinical Interview for DSM-IV.
J Affect Disord.
Table 1: List of genes and SNPs selected for genotyping within their respective chromosomes.
TNFRSF1 rs4285653
TNFRSF1 rs2294630
Table 1 (cont'd List of genes and SNPs selected for genotyping within their respective chromosomes.
Table 1 (cont'd List of genes and SNPs selected for genotyping within their respective chromosomes.
Table 1 (cont'd List of genes and SNPs selected for genotyping within their respective chromosomes.
Table 2: Haplotype comparisons between problem gamblers (PG) with healthy control subjects, modified qui-squared tests, Golden
Helix, SVS v. 7. Only results with p>0.001 are shown 1.
Haplotype Haplotype
Gene Haplotype
Frequency Frequency
Corrected
Odds Ratio 95% CI
p-valued
Controls
rs919741 CAMK2A
GG
0.25
1.73
rs12514354 CAMK2A GA
0.61
0.63
rs12514354 CAMK2A GG
0.12
2.30
rs919740 CAMK2A
AC
0.53
0.66
rs2241695 CAMK2A
CAMK2D CA
0.48
1.89
CAMK2D AA
0.24
1.99
rs10003275 CAMK2D TA
0.29
0.61
rs4834354 CAMK2D AA
0.32
0.64
rs10003275 CAMK2D AG
0.25
1.76
rs4834349 CAMK2D GA
0.25
0.63
rs17446418 CAMK2D AA
0.33
1.63
rs1524998 CAMK2D GA
0.36
0.65
rs17531026 CAMK2D AG
0.24
0.64
rs2293323 CAMK2D CG
0.37
0.66
rs6836139 CAMK2D AA
0.36
0.67
rs7660775 CAMK2D AT
0.69
0.63
rs10515115 CARTPT AG
rs2134655 DRD3
GG
0.43
1.66
rs7633291 DRD3
AA
0.58
0.67
rs6280 DRD3 AC
rs7755078 GRM1
CC
0.27
1.76
rs362854 GRM1 TC
0.24
1.67
rs2648640 GRM5 AC
rs672981 GRM5 CG
1 Genes highlighted in yel ow were significant after FDR correction (p ≤0.001). Genes in bold and italicized were significant at uncorrected p ≤0.001.
Table 2 (Cont'd): Haplotype comparisons between problem gamblers (PG) with healthy control subjects, modified qui-squared tests,
Golden Helix, SVS v. 7. Only results with p>0.001 are shown1.
Haplotype Haplotype
Corrected
Gene Haplotype
Frequency Frequency
p-valued
Odds Ratio 95% CI
Controls
GA
0.36
0.50
25.9
0.56
0.44
0.70
AA
0.15
0.26
25.6
0.50
0.38
0.66
AA
0.33
0.47
23.4
0.57
0.46
0.72
AA
0.14
0.23
19.9
0.53
0.40
0.70
rs2296973 HTR2A CA
0.16
0.26
18.3 1.9E-05 0.0020 0.56
0.42
0.73
rs6314 HTR2A GG
0.55
0.43
17.5 2.9E-05 0.0025 1.61
1.29
2.02
rs6313 HTR2A
GA
0.38
0.49
16.4 5.1E-05 0.0035 0.63
0.50
0.79
rs9534512 HTR2A AC
0.13
0.21
15.2 9.6E-05 0.0050 0.56
0.42
0.75
rs6313 HTR2A
GC
0.20
0.12
14.8 1.2E-04 0.0059 1.90
1.36
2.64
rs9534512 HTR2A CA
0.20
0.12
12.2 4.7E-04 0.0123 1.79
1.29
2.48
rs1923885 HTR2A AG
0.42
0.52
11.9 5.5E-04 0.0133 0.67
0.54
0.84
rs4942577 HTR2A GA
0.28
0.37
10.8 1.0E-03 0.0222 0.67
0.53
0.85
rs7997012 HTR2A GA
10.5 1.2E-03 0.0229 1.57
rs582854 HTR2A AA
10.5 1.2E-03 0.0229 0.62
rs1379170 HTR3A AA
14.0 1.8E-04 0.0074 1.77
10.2 1.4E-03 0.0247 0.69
PLD2
CG
0.49
0.36
23.0
1.75
1.39
2.21
rs2286670 PLD2
CA
0.39
0.49
12.1 5.1E-04 0.0130 0.67
0.54
0.84
rs2286671 PLD2
GA
0.15
0.08
11.0 9.3E-04 0.0207 1.89
1.29
2.76
rs12404263 PRKACB
GA
0.36
0.24
20.2
1.78
1.38
2.29
PRKACB GA
0.42
0.31
15.7 7.5E-05 0.0049 1.61
1.27
2.05
PRKACB AA
0.15
0.08
15.4 8.7E-05 0.0051 2.16
1.46
3.20
PRKACB AG
0.34
0.23
15.4 8.9E-05 0.0049 1.67
1.29
2.16
PRKACB AG
0.40
0.51
14.0 1.8E-04 0.0077 0.65
0.52
0.82
PRKACB GG
0.41
0.51
12.4 4.3E-04 0.0115 0.67
0.53
0.84
1 Genes highlighted in yel ow were significant after FDR correction (p ≤0.001). Genes in bold and italicized were significant at uncorrected p ≤0.001.
Table 2 (Cont'd): Haplotype comparisons between problem gamblers (PG) with healthy control subjects, modified qui-squared tests,
Golden Helix, SVS v. 7. Only results with p>0.001 are shown1.
Haplotype Haplotype
Corrected
Gene Haplotype
Frequency Frequency
p-valued
Odds Ratio 95% CI
Controls
rs140700 SLC6A4
GC
0.12
0.06
13.0 3.0E-04 0.0099
2.19
1.42
3.39
rs17564182 TACR1
CA
0.16
0.23
10.8 1.0E-03 0.0222
0.63
0.48
0.83
rs11688000 TACR1
10.6 1.1E-03 0.0230
10.3 1.3E-03 0.0242
rs2303380 TTC12
AG
0.25
0.17
12.9 3.3E-04 0.0104
1.69
1.27
2.25
1 Genes highlighted in yel ow were significant after FDR correction (p ≤0.001). Genes in bold and italicized were significant at uncorrected p ≤0.001.
Table 3: Haplotype comparisons between problem gamblers with alcohol and/or drug abuse (PG-ADA) with control subjects;
modified qui-squared tests, Golden Helix, SVS v. 7. Association trends with uncorrected p ≤
0.01 are shown1.
Haplotype Haplotype
Corrected
Odds Ratio
Gene Haplotype
Frequency Frequency
χ2 Nominal
p-valued
Controls
GCA 0.16 0.29 10.1
0.001 0.20 0.48 0.30 0.76
HTR2A AGC 0.17 0.08 9.9
0.002 0.18 2.47 1.39 4.37
AGC 0.33 0.20 9.9
0.002 0.17 1.94 1.28 2.93
HTR2A AAG 0.14 0.26 9.4
0.002 0.20 0.48 0.30 0.77
GGG 0.12 0.05 9.3
0.002 0.20 2.89 1.43 5.85
HTR2A AGG 0.14 0.06 9.1
0.003 0.21 2.63 1.38 5.01
HTR2A AGA 0.09 0.03 9.1
0.003 0.20 3.30 1.46 7.45
HTR2A GAA 0.15 0.26 8.7
0.003 0.23 0.50 0.31 0.79
rs6313 HTR2A GCA 0.20 0.11 8.5
0.004 0.24 2.12 1.27 3.53
AGA 0.17 0.28 8.3
0.004 0.26 0.51 0.33 0.81
AAA 0.25 0.15 8.3
0.004 0.25 1.95 1.23 3.08
CAA 0.25 0.15 7.9
0.005 0.28 1.91 1.21 3.01
CNR2 AGA 0.28 0.18 7.8
0.005 0.29 1.86 1.20 2.87
TACR3 ACA 0.11 0.05 7.6
0.006 0.30 2.58 1.29 5.17
AAC 0.22 0.34 7.6
0.006 0.30 0.56 0.37 0.85
TACR3 AGC 0.36 0.25 7.3
0.007 0.34 1.73 1.16 2.57
GGG 0.12 0.05 7.0
0.008 0.37 2.42 1.24 4.73
TTC12 AAA 0.08 0.16 6.9
0.008 0.37 0.45 0.25 0.83
TACR3 GCA 0.36 0.25 6.9
0.009 0.36 1.70 1.14 2.52
TTC12 AAT 0.08 0.16 6.9
0.009 0.35 0.45 0.25 0.83
GCG 0.25 0.16 6.9
0.009 0.35 1.82 1.16 2.85
HTR2A AAA 0.14 0.23 6.7
0.010 0.37 0.53 0.32 0.86
HTR2A AAA 0.14 0.23 6.7
0.010 0.36 0.52 0.32 0.86
TTC12 GAA 0.08 0.16 6.6
0.010 0.38 0.46 0.25 0.84
1. Genes in bold and italicized were significant at uncorrected p ≤0.001
Table 4: Haplotype comparisons between problem gamblers with alcohol and/or drug abuse (PG-ADA) with PG subjects without
substance abuse history; modified qui-squared tests, Golden Helix, SVS v. 7. Association trends with uncorrected p ≤
0.01 are
shown1.
Haplotype Haplotype
Gene Haplotype
Frequency Frequency
Corrected Odds
Odds Ratio
p-valued
Controls
rs7660775 CAMK2D
CAG
0.11
0.24
12.7 0.0004
0.23
0.39
0.23
0.66
rs1011973 CAMK2D GCA
rs3815072 CAMK2D AAA
rs2285704 CAMK2D AAA
rs11098195 CAMK2D AAA
rs17531026 CAMK2D AAA
rs3815072 CAMK2D GAG
rs6533690 CAMK2D GGA
rs17531554 CAMK2D ACG
rs10009286 CAMK2D ACA
rs2293323 CAMK2D CGA
rs2285704 CAMK2D GAA
rs17446418 CAMK2D AGG
rs11098198 CAMK2D AGC
rs13144613 CAMK2D CAC
rs2189364 CAMK2D AAG
rs686 DRD1 GA 0.03 0.08 6.6 0.010 0.36 0.29
rs4648318 DRD2
AGA
0.06
0.17
10.8 0.001
0.42 0.33 0.17 0.66
1 Genes in bold and italicized were significant at uncorrected p ≤0.001
Table 4 (Cont'd): Haplotype comparisons between problem gamblers with alcohol and/or drug abuse (PG-ADA) with PG subjects
without substance abuse history; modified qui-squared tests, Golden Helix, SVS v. 7. Association trends with uncorrected p ≤
0.01
are shown1.
Haplotype Haplotype
Gene Haplotype
Frequency Frequency
Nominal Corrected Odds
Odds Ratio
p-valued
Controls
rs362854 GRM1 TCC
0.07
0.20 12.9 0.0003 0.42 0.33 0.18 0.62
rs7755078 GRM1 CCG
9.2 0.002 0.38 0.47 0.28 0.77
rs1125462 GRM1 ACG
8.2 0.004 0.35 0.30 0.13 0.71
rs7755078 GRM1 ACG
7.0 0.008 0.41 1.79 1.16 2.75
rs672981 GRM5 AGG
0.19 9.8 0.002 0.43 0.39 0.21 0.71
rs2648640 GRM5 GAG
8.4 0.004 0.34 0.41 0.22 0.76
rs11021034 GRM5 GAA
rs1923885 HTR2A AAC
rs9607272 MAPK1 ACA
MAPK1 CA 0.47 0.60 6.9 0.008 0.38 0.60
rs5755099 MAPK1 CCA
rs2294630 NBL1 AGC
7.5 0.006 0.43 1.80 1.18 2.73
rs3771827 TACR1 AAA
rs12641703 TACR3
rs3822292 TACR3 ACA
0.08 8.8 0.003 0.35 0.20 0.06 0.65
rs7927508 TTC12 AAA
9.2 0.002 0.34 0.40 0.21 0.73
rs7130072 TTC12 GAA
8.8 0.003 0.39 0.41 0.22 0.75
1 Genes in bold and italicized were significant at uncorrected p ≤0.001
Figure 6: Diagrammatic Representation of the DRD4 gene and DRD4 exon III VNTR.
Representation of the DRD4 gene and the alleles found in the DRD4 exon III VNTR
polymorphism. Each allele is named after the number of repetitions of a 48bp sequence present
in that allele, i.e. the 4-repeat allele has 4 repetitions of the 48bp sequence. Percentages represent
frequency of the alleles in Caucasian population. (Reproduced with permission from: Ding et al.,
PNAS January 8, 2002 vol. 99 (1): 309-314)
Figure 7: Dopamine D4 receptor graphical representation:
The DRD4 gene synthesizes the D4 receptor. Repeated sequences in the DRD4 gene (more specifically located in the exon III of the gene) are responsible for synthesizing a loop in the receptor (loop synthesized by the 4-repeat allele represented in orange). If the gene has more repeats (e.g. 7-repeat allele), the receptor loop will be longer (loop synthesized by the 7-repeat allele represented in pink).
Figure 8: Screenshot of the sequence alignment (DRD4 exon III)
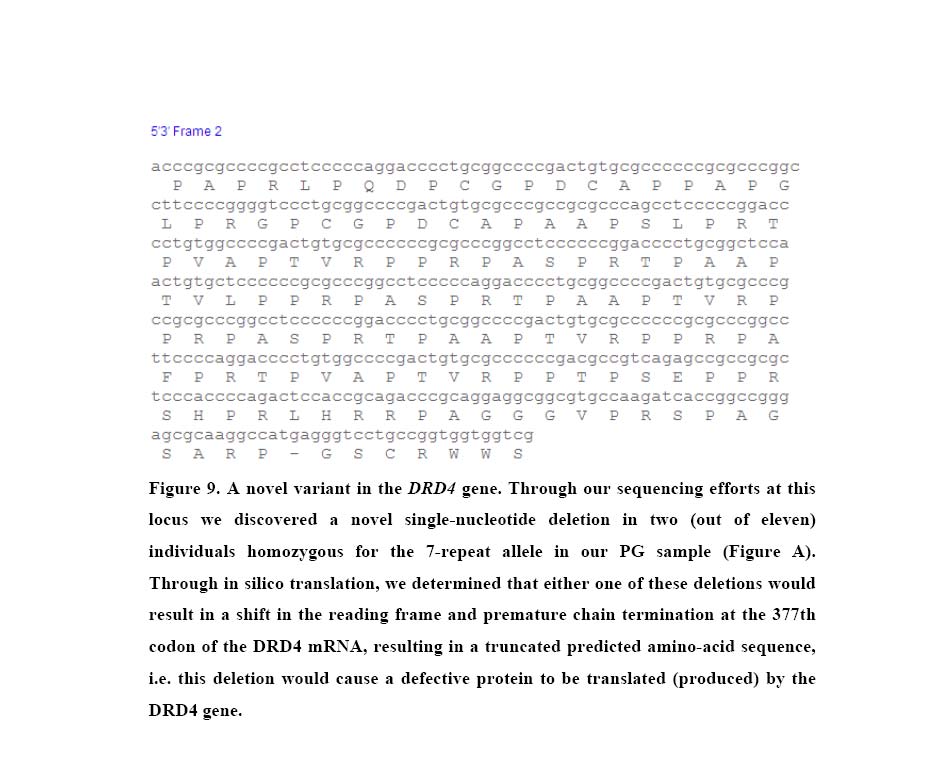
Figure 9: Screenshot of the deletion on DRD4 exon III from one subject in our sample
Source: https://www.problemgambling.ca/EN/Documents/InvestigationofSelectedSignallingSystem%20Genes.pdf
Extended spectrum beta-lactamases A. Beta-lactam antibiotics a. d. Mechanism of resistances B. Beta-lactamases a. b. Extended spectrum beta-lactamases (ESBL) c. Non-TEM, non-SBV ESBL d. Inhibitor Resistant TEM (IRT) C. Definition, classification and properties of ESBL D. Epidemiology and risk factors E. Laboratory detection and identification of ESBLs
NUEVAS FORMAS DE CREAR julio-agosto de 2004 julio-agosto de 2004 Revista de la OMPI/ Revista de la OMPI/ A primera vista, el olor del césped particular de un producto pueden Marcas tridimensionales y Las empresas deben prestar especial recién cortado, el color lila, el grito de adquirir un carácter distintivo y con- formas de productos atención a la descripción y a la repre-