Advances in diagnostic and therapeutic ultrasound imaging
C H A P T E R 3
3-D US Imaging of the Carotid Arteries
Aaron Fenster, Grace Parraga, Anthony Landry, Bernard Chiu, Michaela Egger,and J. David Spence
Determining the severity of carotid atherosclerotic stenosis has been an importantstep in establishing patient management pathways and identifying patients who canbenefit from carotid endarterectomy versus those who should be treated using life-style and pharmaceutical interventions. Recently a number of research groups havedeveloped phenotypes other than carotid stenosis using noninvasive imaging. Mon-itoring carotid plaque progression/regression and identifying vulnerable orhigh-risk plaques that can lead to thrombogenic events using noninvasive imagingtools now involve multiple disciplines and multiple modalities, including imageprocessing.
Morphological characterization of carotid plaques has been used for risk strati-
fication and genetic research [1, 2], evaluation of a patient's response to therapy [3],evaluation of new risk factors [4, 5], and quantification of the effects of new thera-pies [6]. Although 1-D measurements, such as the intima-media thickness, havebeen important for measuring the progression of carotid end-organ disease, 2-Dmeasurements of a plaque area have been shown to have significant advantagesbecause the plaque area progresses more than twice as quickly as does the thickness[4]. The use of conventional ultrasound in measuring plaque size (cross-sectionalarea) in a single longitudinal view has several limitations.
Conventional 2-D US has been used to quantify and correlate plaque morphol-
ogy and composition with the risk of stroke; however, the results have been mixed.
Some investigators have reported high accuracies for identifying features such asintraplaque hemorrhage [7, 8], but others have found only moderate sensitivity andspecificity [9]. Some of these discrepancies are related to the lack of standardizationand the variability of conventional ultrasound exams. However, it is generallyagreed that several ultrasound techniques must be improved in order to more accu-rately and precisely quantify and characterize plaques.
Conventional 2-D US has also been useful in the assessment of plaque mor-
phology. However, due to insufficient image contrast and to the variability of con-
3-D US Imaging of the Carotid Arteries
ventional 2-D US exams, accurate assessments of morphological plaque changes (ofvolume and surface irregularity) are difficult. Because it is difficult to relocatethe 2-D image of the plaque, monitoring the changes in the development of theplaque over long periods of time using conventional 2-D US is problematic. In addi-tion, the reconstruction of a 3-D impression of the vascular anatomy and theplaque using multiple 2-D images is time consuming and prone to variability andinaccuracy [10, 11].
Three-dimensional ultrasound is a recent development that promises to improve
the visualization and quantification of complex anatomy and pathology to monitorthe progression of atherosclerosis [11]. Noninvasive and reproducible 3-D imagingtechniques that allow for the direct visualization and quantification of plaque devel-opment are becoming more important in serial monitoring of disease progressionand regression [12–15]. Furthermore, the characterization of carotid plaques inthree dimensions will potentially improve investigations into the changes of surfacemorphology, plaque geometry, and plaque distribution. These investigations canprovide important information about the effects of antiatherosclerotic therapies.
Limitations of Conventional Carotid Ultrasound Imaging
In conventional carotid ultrasound examinations, the transducer is manipulatedmanually over the arteries by a sonographer or a radiologist. This approach workswell when using ultrasound to
diagnosis or
detect the presence of carotid disease;however, quantitative measurements are subject to variability. Obtaining therequired information from a series of 2-D images is largely dependent on the skilland experience of the operator in performing these tasks. This approach issuboptimal due to the following limitations:
1. Conventional ultrasound images are two dimensional; hence, the
diagnostician must mentally transform multiple images in order toreconstruct a 3-D impression of the complex 3-D structure of the vessel andplaque. This may lead to variable and incorrect quantification, size, andextent of plaque morphology.
2. Conventional 2-D US images are difficult to reproduce; therefore,
conventional 2-D US is nonoptimal for quantitative prospective studies,particularly where small changes in the plaque due to therapy are beingfollowed over the course of time.
3. The patient's anatomy sometimes restricts the angle of the image, resulting in
the inaccessibility of the optimal image plane necessary for the diagnosis ofcarotid disease and the assessment of plaque morphology.
measurements of the volumes of the lesion. Several techniques for improvingthe management of atherosclerosis are now available; however, current 2-DUS volume measuring techniques, which assume an idealized shape of thelesion to calculate the volume, use only simple measures of the width in a fewviews. This method potentially leads to inaccurate results and operatorvariability.
3-D US Imaging of the Carotid Arteries
3-D US Imaging of the Carotid Arteries
Recent research has shown that 3-D US of the carotid arteries not only allows forthe visualization, measurement (volume), and characterization of the carotid plaquebut also provides the capability to monitor plaque progression and regression aswell as to identify vulnerable plaques [13, 16–18]. The ability to monitor the pro-gression and regression (i.e., the changes in volume and morphology) of carotidplaques quantitatively provides researchers with important information about theplaque's response to therapy (e.g., medication or diet mediated lowering of lipids)and the plaque's natural history [15, 18–21].
In the following sections, we review the methods developed and utilized for
acquiring 3-D carotid ultrasound images and the tools required for visualizing andmeasuring plaque volume. The tools for monitoring the changes in the plaque's vol-ume and the plaque's surface features will also be discussed. For further informationabout the technical and computational aspects of 3-D US, readers should refer toChapter 1 of this book and to the recent review articles and books on the subject[11, 22–28].
3-D Carotid Ultrasound Scanning Techniques
Because images of the carotid arteries require at least a 4-cm scanning length,real-time 3-D (four-dimensional) systems cannot be used effectively. Thus, all 3-DUS systems that are currently used to acquire images of the carotid arteries are con-ventional 1-D US transducers that produce 2-D US images. Different methods areused to locate the positions and orientations of 2-D images within the volume. Two3-D US approaches have been successfully used to image the carotid arteries:mechanical linear scanners and magnetically tracked free-hand scanners. Theseapproaches are summarized in the following sections.
Mechanical Linear 3-D Carotid Ultrasound Imaging
Linear scanners use a motorized mechanism to translate the transducer linearlyalong the neck of the patient as shown in Figure 3.1. While the transducer is moved,transverse 2-D images of the carotid arteries are acquired at regular spatial inter-vals. Each image in the set of acquired 2-D images is spaced equally so that all subse-quent images are uniform and parallel to the preceding image. The length of thescan, which is the image length of the carotid arteries in 3-D, depends on the lengthof the mechanical scanning mechanism. The resolution of the image in the 3-D scan-ning direction (i.e., along the artery) can be optimized by varying the translatingspeed and sampling interval in order to match the sampling rate to the frame rate ofthe ultrasound machine and to match the sampling interval to (half) the elevationalresolution of the transducer [29]. Typically, 2-D US images are acquired every 0.2mm. If the 2-D US images are acquired at 30 frames per second, a 4-cm length willrequire 200 2-D images, which can be scanned in 6.7 seconds without cardiacgating.
The simple predefined geometry of the acquired 2-D US images can be recon-
structed to form a 3-D image [29]. Using this approach, a 3-D image can be viewed
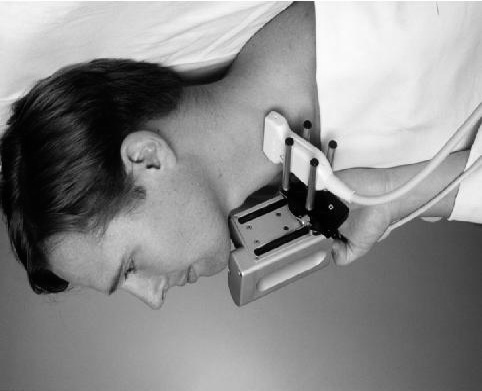
3-D US Imaging of the Carotid Arteries
Figure 3.1
Photograph of a mechanical linear scanner mechanism used to acquire 3-D US
images of the carotid arteries. The transducer is translated along the arteries, while conventional2-D US images are acquired by a computer and reconstructed into a 3-D image.
immediately after scanning to determine if additional 3-D scans are necessary. Thespecific advantages of immediate review of 3-D images after a scan are that it signifi-cantly shortens the examination time and reduces digital storage requirementsbecause a decision can be made not to store unnecessary 3-D images.
Because the 3-D carotid ultrasound image is produced from a series of conven-
tional 2-D images, the resolution in the 3-D image will not be isotropic. In the direc-tion parallel to the acquired 2-D US image planes, the resolution of thereconstructed 3-D image will be equal to the original 2-D images; however, in thedirection of the 3-D scan along the arteries, the resolution of the reconstructed 3-Dimage will depend on the elevational resolution of the transducer and the interslicespacing [29]. Because the elevational resolution is less than the in-plane resolution ofthe 2-D US images, the resolution of the 3-D US image will be the lowest in the 3-Dscanning direction (i.e., elevation). Therefore, a transducer with good elevationalresolution should be used to obtain optimal results.
The scanning parameters can be adjusted depending on the following two types
of ultrasound images: 3-D B-mode and 3-D power Doppler. The 3-D B-mode is usedfor imaging vessel walls and plaque; one to three focal zones result in a 2-D imageacquisition rate of about 30 to 15 frames per second and a 3-D scan of 6.7 to 13.4seconds for the acquisition of 200 2-D US images. For 3-D power Doppler imaging,which is used to display blood flow, an increased persistence with no cardiac gatingresults in an acquisition rate of 7.5 images per second and a 3-D scan time of 20seconds.
The 3-D mechanical scanning offers three advantages: short imaging times,
high-quality 3-D images, and fast reconstruction times. However, its bulkiness andweight sometimes make it inconvenient to use. Linear scanning has been successfullyimplemented in many vascular imaging applications using B-mode and color Dopp-ler images of the carotid arteries [30–34], vascular test phantoms [35–37], andpower Doppler images [30–36]. An example of a mechanical scanner is shown inFigure 3.1, and several examples of linearly scanned 3-D US images of carotid arter-ies with complex plaques are shown in Figure 3.2.
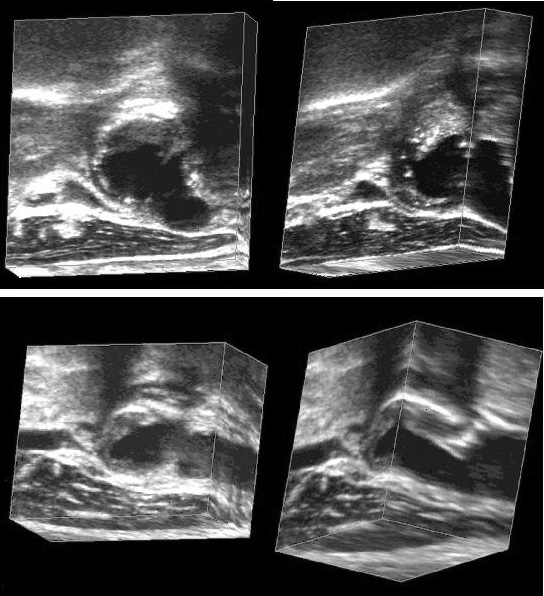
3-D US Imaging of the Carotid Arteries
Figure 3.2
Two 3-D US views of two different patients with complex and ulcerated carotid
plaques. For each patient, (a, c) a transverse and (b, d) a longitudinal view are shown side by side.
Magnetically Tracked Free-Hand 3-D Carotid Ultrasound Imaging
To overcome size and weight issues related to mechanical scanning, free-hand scan-ning techniques have been developed that do not require motorized mechanisms[37–42]. To measure position and orientation, a sensor is attached to the trans-ducer. As the transducer is manipulated across the neck, a set of transverse 2-Dimages of the carotid arteries is digitally acquired by a computer, and the positionand orientation of each image is obtained and recorded. This information is thenused to reconstruct the 3-D image. Because the relative positions of the acquired2-D images are not predefined, the operator must ensure that the set of 2-D imagesdoes not have any significant gaps and that the desired region is scanned. In addi-tion, the ability to scan the neck freely allows longer regions of the neck to beimaged.
Although several free-hand scanning approaches have been developed (e.g.,
articulated arms, acoustic sensing, magnetic field sensing, and image-based sens-ing), the most successful approach for imaging the carotid arteries uses a sensorwith six degrees of freedom to measure the strength of a magnetic field. In thisapproach, a transmitter is used to produce a spatially varying magnetic field; a smallsensor detects the magnetic field in order to determine the relative position and ori-
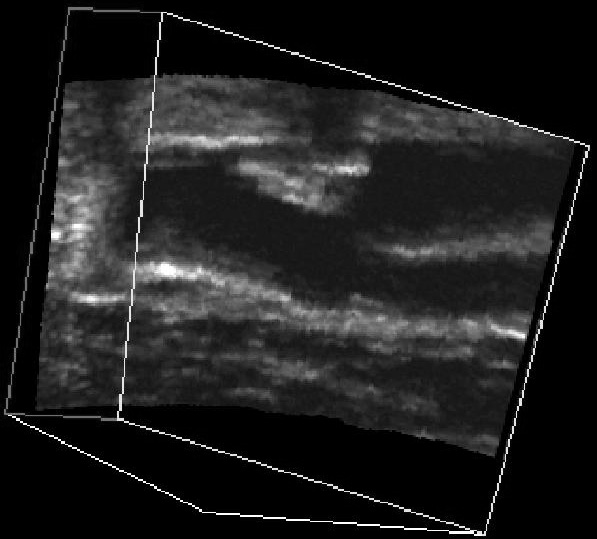
3-D US Imaging of the Carotid Arteries
entation of the sensor. Typically, the transmitter is placed beside the patient and thesensor is mounted onto the transducer.
The magnetic field sensors are small and unobtrusive devices that allow the
transducer to be tracked with fewer constraints than any other mechanical scanningapproach. However, electromagnetic interference (e.g., ac power cabling andmotors) can compromise the accuracy of tracking. Geometric distortions in the final3-D US image can occur if ferrous or highly conductive metals are located nearby.
By ensuring that the environment near the patient is free of metals and electricalinterference, high-quality 3-D images of the carotid arteries can be produced. Figure3.3 shows a 3-D carotid image that has been obtained with a magnetic field sensor.
Reconstruction of 3-D US Images
In the 3-D reconstruction procedure, the set of acquired 2-D images is used to recon-struct a 3-D (voxel-based) volume. Each of the 2-D US images is placed in its correctlocation within the volume. The gray-scale values of any volume element (or voxel)not sampled by the 2-D images are then calculated by interpolating between theappropriate images. As a result, all 2-D image information is preserved, allowing forthe viewing of the original 2-D planes as well as the other types of views. The adventof desktop computers has made this process very efficient by allowing 3-D recon-structions to occur while 2-D images are being acquired. Thus, it is possible to view
Figure 3.3
A 3-D B-mode ultrasound image of carotid arteries obtained with the free-hand scan-
ning approach using a magnetic position and orientation measurement. The 3-D image has been"sliced" to reveal a plaque at the entrance of the internal carotid artery. The plaque isheterogenous, with calcified regions casting a shadow.
Measurement of Plaque Volume
the complete 3-D US image immediately after the acquisition of the images has beencompleted.
Viewing of 3-D US Images
After a 3-D image has been reconstructed, it is ready to be viewed. The cube-viewapproach is based on multiplanar rendering using texture mapping. In this tech-nique, a 3-D image is displayed as a polyhedron, and the appropriate image for eachplane is "painted" on the face of the cube (texture mapped). Users not only canrotate the polyhedron in order to obtain the desired orientation of the 3-D image,but also can move any of the surfaces (i.e., by slicing of the 3-D image parallel orobliquely) to the original, while the appropriate data are texture mapped in realtime onto the new face. As a result, users always have 3-D image-based cues, whichrelate the plane being manipulated to the rest of the anatomy. These visual cuesallow users to efficiently identify the desired structures [11, 22, 26, 27]. Examples ofthis approach are shown in Figures 3.2 and 3.3.
In the orthogonal plane view, three perpendicular planes are extracted from the
volume data and displayed simultaneously on a computer screen as 2-D images.
Graphical cues are added to the screen and overlaid onto the 2-D images to indicatetheir relative orientations. Users can then select and move single or multiple planeswithin the 3-D image volume to provide a cross-sectional view at any location ororientation, including oblique views.
The volume rendering technique presents a display of the entire 3-D image after
it has been projected onto a 2-D plane. The projection of the image is typicallyaccomplished via ray-casting techniques [43]. The image voxels that intersect eachray are weighted and summed. The desired result is displayed in the rendered image.
Although this approach has been used primarily to display fetal anatomy, it can alsobe used to display vascular anatomy [30] and views of carotid plaques, as shown inFigure 3.4.
Measurement of Plaque Volume
Plaque Segmentation by Manual Planimetry
Carotid disease can be measured using either of two manual planimetry techniques:the plaque volume technique or the vessel wall volume technique. When using theplaque volume technique, each 3-D image is "sliced" transverse to the vessel axis,starting from one end of the plaque using an interslice difference (ISD) of 1.0 mm[Figure 3.5(a)]. Using software developed in our laboratory, we can contour theplaque in each cross-sectional image using a cross-haired cursor (Figure 3.5). As thecontours are manually outlined, the visualization software calculates the area of thecontours automatically. Sequential areas of the contours are averaged and multi-plied by the ISD in order to calculate the incremental volume. A summation ofincremental volumes provides a measure of the total plaque volume. After measur-ing a complete plaque volume, the 3-D US image can be viewed in multiple orienta-tions in order to verify that the set of contours has outlined the entire plaque
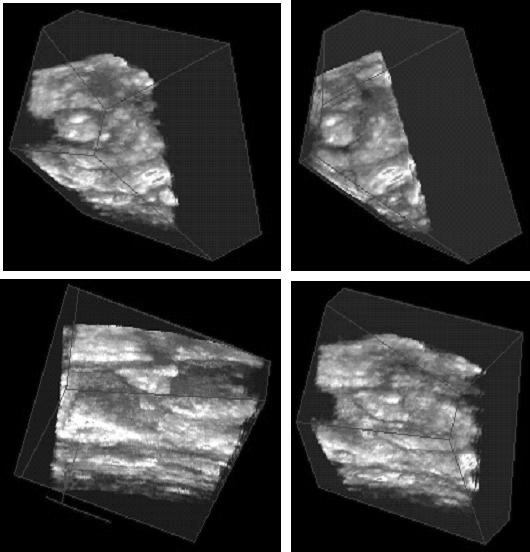
3-D US Imaging of the Carotid Arteries
Figure 3.4
Four views of the 3-D carotid ultrasound image shown in Figure 3.3. Each panel has
been volume rendered to display the appearance of the plaque in the common carotid artery. Thisapproach enhances the appearance of the plaque as image speckle is suppressed.
volume. A typical plaque having 10 to 30 slices requires approximately 15 minutesof measuring time.
The vessel wall volume technique, which is commonly used for analyzing MR
images, is an alternative method for quantifying disease in the carotid arteries. Inthis approach, the lumen (blood–intima boundary) and the vessel wall(intima–adventia boundary) are segmented in each slice. The area inside the lumenboundary is subtracted from the area inside the vessel wall boundary. Sequentialareas are averaged and multiplied by the ISD to give the incremental vessel wall vol-ume. The summation of incremental volumes provides a measure of the total vesselwall volume (Figure 3.6).
Studies of Plaque Volume
During the past few years, a number of investigators have explored the use of 3-DUS imaging in measurements of carotid plaque volume [15, 44–47]. These in vivoand in vitro investigations have also reported on the variability of plaque volumemeasurements for plaques ranging in size and geometry. Delcker et al. [45–49] havemeasured carotid plaque volumes ranging from 2 to 200 mm3. The intraobserverand interobserver variability for the full series of plaque sizes were found to be 2.8%and 3.8%, respectively, demonstrating that 3-D US imaging can be used to obtainreproducible measurements of carotid plaque volumes [36, 45, 49]. Delcker et al.
have further demonstrated that in vivo measurements of carotid plaques have bene-
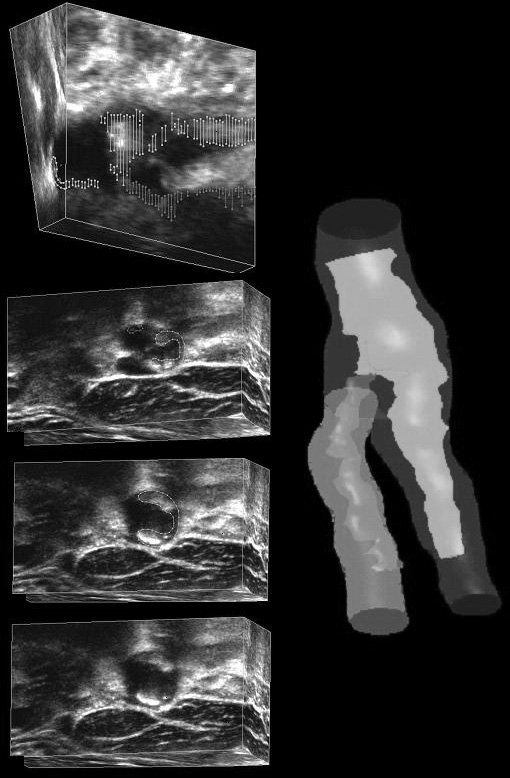
Measurement of Plaque Volume
Figure 3.5
Steps used in measurement of total plaque volume from 3-D US images. (a) First, the
3-D image is "sliced" to obtain a transverse view. (b, c) Using a mouse-driven cross-haired cursor,the plaque is outline in successive image "slices" until all the plaques have been traversed. (d) Thevessel can be sliced to reveal a longitudinal view with the outlines of the plaques. (e) After outlin-ing all of the plaques, the total volume can be calculated and a mesh fitted to provides a view ofthe plaque surface together with the boundary of the vessel.
fitted from ECG-triggered data acquisition. The intraobserver variability, theinterobserver variability, and the follow-up variability were shown to decrease sig-nificantly when compared to the non-ECG-triggered measurements [49]. Palomboet al. [47] measured carotid plaque volume ranging from 7 to 450 mm3 andobtained reliability coefficients that were close to 1 for this in vivo intraobserverand interobserver study.
The monitoring of carotid plaque progression and regression requires accurate
and reproducible techniques to measure and analyze carotid plaque volume andmorphology. Thus, reproducible segmentations of the vessel lumen, plaque surface,and carotid wall borders are required. Although a number of studies have demon-strated the potential utility of 3-D US, few have attempted to quantify the accuracy
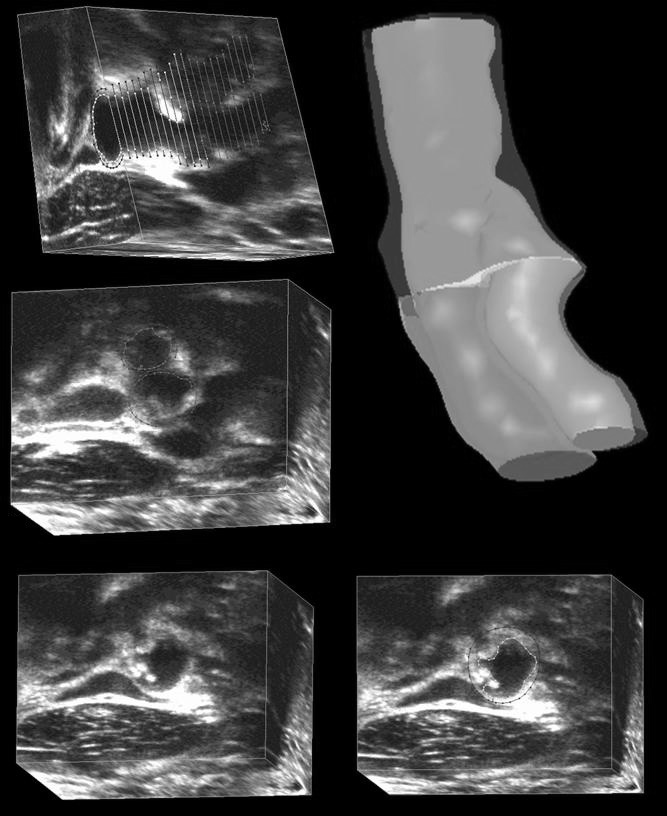
3-D US Imaging of the Carotid Arteries
Figure 3.6
Steps used in measurement of vessel wall plus plaque volume from 3-D US images.
(a) First, the 3-D image is "sliced" to obtain a transverse view. (b, c) Using a mouse-drivencross-haired cursor, the vessel boundary and the lumen boundary plaque are outlined separately insuccessive image "slices" until all the slices have been traversed (typically 1.5 cm above and belowthe carotid bifurcation). (d) The vessel can be sliced to reveal a longitudinal view with the outlinesand correct any errors. (e) After outlining has been completed, the vessel wall plus plaque volumecan be calculated and a mesh fitted to provide a view of the vessel and the lumen boundaries.
of these measurements by determining the actual volume of each plaque. In addi-tion, the variability in the measurement of plaque volume has generally beenreported over a large range of plaque volumes. In the analysis of plaque progression,it is important to understand the relationship between plaque volume and plaquevolume measurement variability so that plaque changes at different stages of plaqueprogression can be interpreted appropriately. In the following sections we review
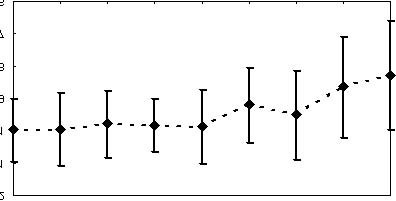
Measurement of Plaque Volume
both the accuracy and the variability of plaque measurement as a function of plaquevolume.
The Effects of Interslice Distance
The value of the ISD between the slices of the acquired 3-D US images will affect themeasurement of the plaque volume. If the value of the ISD is chosen to be small (inorder to obtain results that are accurate), then the quantification of the volume pro-cedure will be time consuming. If the value of ISD is chosen to be large (in order toreduce the number of analyzed slices and to reduce the time of measurement), theninaccurate and variable results may occur. Thus, we investigated the effects of theISD on the relative accuracy and variability of the plaque volume measurement bymeasuring the volume of five plaques, which ranged in size from 42.2 to 604.1 mm3.
These plaques were measured five times using nine separate ISDs, which rangedfrom 1.0 to 5.0 mm in increments of 0.5 mm. The results are shown in Figure 3.7.
These results show that the relative plaque volume (normalized to measurementswith an ISD of 1 mm) had remained unchanged for ISDs between 1.0 and 3.0 mmand had decreased to 0.83 mm for larger ISDs. Thus, the plaque volume will be sys-tematically underestimated for ISDs greater than 3.0 mm. The error bars in Figure3.7 show that the variability of the plaque volume measurement increases as the ISDincreases. The coefficient of variation (i.e., the SD divided by the mean), whichincreased as the ISD increased, was found to be approximately 10% for ISDs of 3mm or less. Based on these results, we had chosen an ISD of 1 mm for quantifyingplaque volume.
The Accuracy of Plaque Volume Measurements
To ascertain the accuracy of the plaque volume measurements, the volume of themeasured plaque must be compared to the actual volume of the plaque. Because 3-DUS-based measurements of carotid plaques in vivo cannot be compared to the actualplaque volume obtained by any other method, an in vitro study using test phantomswith known "plaque" volumes was conducted by Landry and Fenster [50]. In this in
Inter-slice distance (mm)
Figure 3.7
Relative mean plaque volume as a function of ISD for five plaque volumes measured
by a single observer five times using each ISD investigated. Relative volume is constant for ISDs of1.0 to 3.0 mm, but then decreases to 0.83 mm for an ISD of 5.0 mm. Plaque volume measure-ment variability is shown by the error bars that represent ±1 SD. (From: [51]. 2004 AmericanHeart Association. Reprinted with permission.)
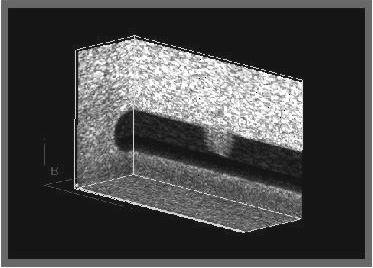
3-D US Imaging of the Carotid Arteries
vitro study, the vascular phantoms were made from blocks of colloidal agar thatcontained Sigmacell to scatter the ultrasound beam and to produce the typical ultra-sound image speckle; cylindrical channels were used to simulate the vessels. Theagar gel was sliced into "plaques" of varying height and length in order to produceseveral simulated hypoechoic plaques. These "plaques" were then inserted into thecylindrical channels, imaged with 3-D US, and measured.
The results from this study are shown in Figure 3.8. The measurement error of
the plaque volume is plotted as a function of plaque volume, which ranges from 68.2to 285.5 mm3. The inserted image is a 3-D US sample of the simulated plaque in thevascular phantom. The mean error for the measurement of the plaque volume wasfound to be 3.1% ± 0.9%. The coefficient of variation (i.e., the SD divided by themean) in the measurement of the plaque volume was found to be 4.0% ± 1.0% and5.1% ± 1.4% for intraobserver and interobserver measurements, respectively. Usingthe manual planimetry method, Landry and Fenster have developed a theoreticaldescription for the variance in the measurement of plaque volume [50]. Theroot-mean-square (RMS) difference between the experimentally and the theoreti-cally determined values of the coefficient of variation for plaque volumemeasurement was found to be 9%.
Variability of Plaque Volume Measurements
The variability of plaque volume measurements has been reported by a number ofinvestigators as an average that has been calculated over a wide range of plaque vol-
Figure 3.8
(a) The error in the simulated plaque volume measurement plotted as the absolute
percent difference between the actual (V ) and measured (V ) plaque volumes as a function of the
average actual volume for each plaque. The error bars represent ±1 SD. (b) A longitudinal viewof the 3-D image of the simulated vessel and plaque sliced to reveal the simulated vessel (black)and plaque. (From: [50]. 2002 American Association of Physicists in Medicine. Reprinted withpermission.)
Measurement of Plaque Volume
umes; however, it is also important to assess the effect of plaque volume on the vari-ability in the measurement of plaque volume. To examine and determine this effect,we conducted a multiple observer study, which determined the intraobserver andinterobserver variability in the measurement of plaque volume. Each of the fiveobservers measured the volume of 40 carotid plaques five times during five differentsessions using an ISD of 1.0 mm. Using a 3-D mechanical scanning approach, weimaged the carotid plaques from a number of human subjects (23 males and 17females with an average age of 73.8 ± 6.2 years) who were patients at the PrematureAtherosclerosis Clinic and the Stroke Prevention Clinic (London Health ScienceCentre, University Campus, London, Ontario, Canada). The volume of the plaquesranged from 37.4 to 604.1 mm3.
Table 3.1 and Figure 3.9 show a summary of the results obtained using Analysis
of Variance (ANOVA) [52, 53]. Table 3.1 lists the results for the entire set of data aswell as for the five subsets (a, b, c, d, e) consisting of seven to eight plaques, whichwere grouped together by volume.
For the smallest volumes of plaques, which range from 37.4 to 89.5 mm3, Table
3.1 shows the interstandard and intrastandard error of measurement (SEM ) to be
13.1% ± 1.8% and 12.5% ± 1.9%, respectively (subset a). For the largest volumesof plaques, which range from 519.4 to 604.1 mm3 (subset e), the SEM
were found to be 4.3% ± 1.0% and 3.9% ± 1.3%, respectively. Figure 3.9
not only shows this effect but also shows that the coefficient of variation in theintraobserver measurement of the plaque volume decreases as the plaque volumeincreases. For the entire range of plaque volumes measured in this study (37.4 to604.1 mm3), we determined the intraobserver coefficient of variation to be 19.2%and 1.9%, respectively. For the same range of plaque volumes, we determined therange of the interobserver coefficient of variation to be from 24.1% to 2.2%.
Automated Segmentation of Plaques from 3-D US Images
The manual planimetry method for measuring plaque volume requires that an oper-ator: (1) "cut" into a 3-D US image, (2) orient the revealed plane appropriately, and(3) outline the plaque surface manually. To obtain the volume of the completeplaque, the operator must "cut" further into the volume and repeat the process until
Table 3.1
Summary of the ANOVA Results of Study in Which
the Volumes of 40 Plaques Were Repeatedly Measured by FiveObservers
Plaque Subset Mean V (mm3) SEM
/V (%) SEM
Global data
The plaques have been grouped in five subsets, and the mean of each subset tabu-lated. The interobserver and intraobserver coefficient of variation (standard errorof measurement divided by the mean) has been tabulated.
Source: [51].
3-D US Imaging of the Carotid Arteries
/(Vσ v 10
Figure 3.9
The results of the ANOVA analysis of the repeated measurements of 40 plaques made
by five observers. The results are plotted as the intraobserver coefficient of variation (standard devi-ation divided by the mean plaque volume), σ /V, as a function of mean plaque volume, V (dia-
monds). The five plaque volumes measured in the repeat 3-D US scan study are plotted as opendiamonds. The error bars represent ±1 SD. (From: [51]. 2004 American Heart Association.
Reprinted with permission.)
the complete plaque is traversed. This approach for manually outlining the plaque isnot only arduous but also time consuming. Substantial experience is needed by anoperator in order to obtain reasonable results.
We have developed an efficient semiautomated segmentation algorithm that is
based on deformable models that require minimal user interaction and processingtime to segment the boundary of plaques [54–57]. Our approach is based on adeformable model that defines a boundary as a series of vertices connected by a setof vectors, which produces a 3-D mesh. This structure allows for the modeling offorces between the nodes of the mesh and the external forces acting on the mesh,which are based on the image properties (e.g., the image gradients found at thelumen boundary). Using this model, we can calculate the forces at each vertex,which is moved iteratively until the values of the forces acting on the vertices arezero.
The algorithm contains three of the following major steps: (1) the placement of
the initial deformable balloon model (i.e., a 3-D mesh composed of triangles) insidethe lumen; (2) the inflation of the model toward the plaque and vessel surface; and(3) the automatic localization of the plaque and vessel surface. When equilibrium isreached (under the influence of inflation forces, internal forces, and image forces),the model approximately represents the shape of the surface. Because the surface ofthe plaque may not be smooth and uniform, we deform the mesh further by using thelocal image-based forces to localize the surface of the plaque. Equations (3.1) and(3.2) describe the dynamics of the model and the forces acting on the model. Equa-tion (3.1) defines the motion for the ith vertex of the mesh:
x ′(t) + g(x (t)) = f(x (t
Measurement of Plaque Volume
The position and velocity of the vertex are given by x (t) and x ′ t
( ), respectively.
The resultant surface tension at the vertex and the "driving" force are given byg(x (t)) and f(x (t)), respectively. Equilibrium is reached when x ′ t
( ) becomes zero.
The equilibrium position of each vertex, and hence the final shape of the model,
is obtained by iteratively updating the variable of time in (3.1) by Δt. Once the sur-face of the plaque has been segmented, an image-based force replaces the inflationforce. The image force causes the mesh to fit the fine details of the plaque. A 3-Dpotential function, P, is constructed from the image data, which attracts the modelto the 3-D gradients at the surface of the plaque:
P x (t)) = 1 [ ∇G * I ε
where Gσ is a 3-D Gaussian smoothing kernel of width σ, and ε is a constant thatprevents the denominator of (3.2) from dividing by zero. The minima of the poten-tial function coincide with the plaque surface. The potential function produces aforce field, which is used to deform the model as f(x (t) = −k P(x (t). The value of k,
which must be optimized so that the mesh will conform to the surface of the plaque,is an adjustable term that controls the strength of the force. In addition, the surfacetension of the mesh must be also chosen appropriately. If the model is too stiff, thenit will not deform into small ulcerations, but if it is too compliant, then the modelmay extend into echolucent regions.
Figure 3.10 shows the results of segmentation using a 3-D image of a patient's
carotid arteries with plaque. The segmentation required only about 10 seconds togenerate the lumen surface of the arteries. The mean separation between manualsegmentation and semiautomated (algorithm) segmentation was 0.35 mm with astandard deviation of 0.25 mm [56].
Figure 3.10
Analysis results of the 3-D carotid segmentation. (a) Image showing local agreement
between manual and algorithm segmentation. Agreement is shown in white and disagreement ingray. (b) Local standard deviation (variability) in the 3-D algorithm segmented boundary, with thescale on the right. (From: [56]. 2000 American Association of Physicists in Medicine. Reprintedwith permission.)
3-D US Imaging of the Carotid Arteries
Plaque Surface Morphology
The use of conventional 2-D US to identify characteristics of the plaque–lumenboundary (i.e., surface irregularities, roughness, and ulcerations) remains difficult.
However, mounting evidence has not only been showing the importance of plaquesurface morphology [58–61] but also generating interest in the development ofimproved ultrasound techniques for visualization and characterizing of plaque sur-face features. Using 3-D US images, we have been developing improved quantitativetechniques for the analysis of plaque surface morphology. Our approach uses thesurface of an outlined plaque, which is obtained by either using manual planimetryor using an automated approach. The outlines of a plaque are then converted to atriangular surface mesh, which is smoothed to remove the variation in the surfacedue to ultrasound speckle. We analyzed the mesh by calculating the local Gaussiancurvature at each node of the mesh. To reduce the variation in the measure of thecurvature, we determined the local mean Gaussian curvature value [62–64] by aver-aging the curvature values of all surface points within a 1-mm radius of each pointon the mesh.
Figure 3.11 shows a result of the surface roughness metric measured by the
mean Gaussian curvature. The roughness metric has been coded in gray and mappedonto the segmented surfaces of the vessels, showing an increase in the roughness forsome regions of the surface associated with the plaque. Although these types of
Figure 3.11
Result of analysis of the surface morphology of a section of a common carotid artery.
The roughness index is coded in gray and is mapped onto the lumen wall–plaque boundary.
Measurement of Plaque Volume
images have yet to be validated, they show the potential of using 3-D US images formonitoring changes in the plaque surface morphology.
Local Distribution and Change of Carotid Wall and Plaque
A number of investigators have studied the spatial distribution of carotid plaque byquantifying the degree of stenosis [20, 65]. Although stenosis profiles are useful indescribing the distribution of plaque along a vessel, they do not give the location ofthe plaque burden within a given transverse slice of the vessel. The following sec-tions describe a metric that can be used to compute the local distribution of carotiddisease on a point-by-point basis for each transverse carotid image slice. In addi-tion, we have extended the quantification of plaque burden along the vessel to thequantification of the change of the vessel wall plus plaque thickness of the carotidvessel between two time points.
Reconstructing Surfaces from Polygonal Slices
Figures 3.12(a) and (c) show the carotid wall and lumen, which were segmentedmanually five times by an expert observer, in 2-D transverse image slices that are 1mm apart. The symmetric correspondence algorithm [66] was used to pair the verti-ces on adjacent contours. With all of the correspondence vertices defined, the adja-cent 2-D curves were connected to form polygonal surfaces. Figure 3.12(b) showsthe tessellated surface, which was constructed from the stack of 2-D contours of thecarotid wall. Figure 3.12(d) shows the surface constructed for the set of contoursrepresenting the carotid lumen.
3-D Maps of Average and Standard Deviation Carotid Surfaces
For each of the 3-D carotid US images, an expert observer segmented the carotidwall and lumen five times. After the five surfaces representing the wall and thelumen had been reconstructed using the method described earlier, we obtained theaverage and standard deviations of the wall and the lumen surfaces using the fol-lowing steps:
1. For the segmentation of the vessel, the transverse plane was identified, and
the average of the normals of the five different transverse planes wascalculated. The five segmented surfaces were then resliced at 1-mm intervalsusing the plane with the average normal, producing five 2-D contours at1-mm intervals along the vessel.
2. Because the symmetric correspondence algorithm only established a
correspondence between a pair of curves, it was applied four times. Thesmoothest curve (of the five curves obtained in step 1) was chosen, andthe symmetric correspondence mappings were established between thesmoothest curve and the remaining four curves.
3. Each of the vertices of the smoothest curve (defined in step 2) was linked
with correspondence points on four different curves, resulting in a group of
3-D US Imaging of the Carotid Arteries
Standard deviation (mm)
Standard deviation (mm)
0.000 0.296 0.592 0.887 1.18
0.000 0.296 0.592 0.887 1.18
Standard deviation (mm)
Standard deviation (mm)
0.000 0.296 0.592 0.887 1.18
0.000 0.296 0.592 0.887 1.18
Figure 3.12
(a) Manually segmented carotid artery wall contours. (b) Mean carotid artery wall
surface obtained with five manual segmentations. The standard deviation at each location is codedin gray and mapped onto the mean surface. (c) Manually segmented carotid artery lumen con-tours. (d) Mean carotid artery lumen surface obtained with five manual segmentations. The stan-dard deviation at each location is color coded and mapped onto the mean surface.
five points. The centroid and the standard deviation of the points about thiscentroid were then computed.
Vessel Wall Plus Plaque Thickness Map and Its Standard Deviation
A transverse plane was used to slice the average surfaces of the carotid wall andlumen at 1-mm intervals. The vertices of the resulting 2-D curves were pairedaccording to the symmetric correspondence algorithm [66]. The 3-D thickness mapwas then computed as the difference between the carotid wall and lumen boundary,which was composed of a stack of 2-D curves, each lying on a transverse planewhere the average surfaces of the wall and the lumen were sliced. As shown in Figure3.13, the resulting thickness map was coded in gray and superimposed on the meanvessel wall 3-D boundary. Figure 3.13 shows the vessel wall plus plaque thickness
Measurement of Plaque Volume
maps for a patient scanned at baseline and after a 3-month interval, during which hewas treated with 80 mg of atorvastatin.
3-D Maps of Carotid Wall Plus Plaque Thickness Change
To study carotid plaque change, we generated the vessel wall plus plaque thicknessmap from 3-D US images of carotid arteries obtained at two different times (seeFigures 3.13 and 3.14). To calculate the change in the plaque thickness between theimaging times, we first registered the vessel contours using a slightly modified ver-sion of the iterative closest point (ICP) algorithm by Besl et al. [67]. Rather thanaligning the centroids of the two surfaces, as proposed in [67], we aligned the bifur-cation apex of the carotid vessels. After registering the two thickness maps, we then
0.000 2.28 4.55 6.83 9.1 0.000 2.28 4.55
Figure 3.13
(a) Mean carotid artery wall with thickness of vessel wall plus plaque is coded in gray
and mapped on its surface (obtained at baseline). (b) The thickness map in part (a) is showntogether with the lumen of the carotid artery. (c) Mean carotid artery wall with thickness of vesselwall plus plaque is coded in gray and mapped on its surface (obtained after 3 months of 80 mg ofatorvastatin daily treatment). (d) The thickness map in part (c) is shown together with the lumenof the carotid artery.
3-D US Imaging of the Carotid Arteries
Figure 3.14
The carotid arteries have been cut open and flattened and the thickness of vessel
wall plus plaque is coded in gray and mapped on its surface. (a) Results obtained at baseline. (b)Results obtained after 3 months of 80 mg of atorvastatin daily treatment.
calculated the difference between them, coded it in gray, and mapped it onto a mean3-D boundary that was obtained from the 3-D US image at baseline, as shown inFigure 3.15.
Figure 3.13 shows the results for a patient who was treated with 80 mg ofatorvastatin daily for 3 months. The results shown in Figure 3.13(a, b) wereobtained at baseline, and the results in Figure 3.13(c, d) were obtained after 3months of treatment. The difference in plaque in the vessel wall plus plaque thick-ness is also apparent in the flattened map of the carotid arteries shown in Figure3.14. The difference in the vessel wall plus plaque thickness is shown in Figure 3.15,which shows a pronounced regression on the left side of the CCA with a reduction ofplaque thickness of about 7 mm.
The calculation and the display of the distribution of carotid plaque and the dis-
tribution of plaque change will help in the study of the natural progression of carotidplaque; more importantly, it will help in the understanding of plaque regression inresponse to therapy.
Trends and Future Developments
Thickness change (mm)
Figure 3.15
(a) The thickness of the vessel wall plus plaque difference between the results
obtained at baseline and after atorvastatin treatment (maps shown in Figure 3.14). A negativevalue indicates that a plaque thickness reduction was recorded in the second scanning session. (b)The flattened map of the 3-D results shown in part (a).
Trends and Future Developments
Three-dimensional ultrasound has already demonstrated its clear advantages inobstetrics (e.g., imaging of the fetus), cardiology (e.g., imaging of the cardiac sys-tem), and image guidance (e.g., imaging of the prostate during an interventionalprocedure) [11, 26, 27]. Advances in 3-D US instrumentation and visualization soft-ware have advanced sufficiently enough to allow for fast (real-time) 3-D acquisi-tion, real-time reconstruction, and 3-D visualization with real-time imagemanipulation. Technical advances continue to improve both 3-D US image acquisi-tion and image analysis, promising to make it a routinely available tool on allultrasound machines.
The availability of improved 3-D US systems is allowing researchers to focus on
demonstrating its clinical utility. Although 3-D US has been shown to be useful inimaging the plaques of the carotid arteries, this modality requires further develop-ment in order for it to become a routine tool for quantifying the progression andregression of carotid disease. The following four sections discuss the current trendsand needed developments in 3-D carotid ultrasound imaging.
3-D US Imaging of the Carotid Arteries
The Need for Improved Free-Hand Scanning
Although free-hand scanning techniques are useful, they are still subject to potentialartifacts and inaccuracies. Further technical progress is needed in order for thesetechniques to become standard tools for producing high-quality images of thecarotid arteries. Approaches for gating and techniques for ensuring that the carotidarteries are imaged uniformly need improvement. Real-time 3-D US systems (using2-D arrays) are useful in cardiology. However, further developments are needed inorder for them to produce 3-D images of the carotid arteries with an image qualitysimilar to the quality that is currently being produced by conventional 1-D arrays.
The Need for Improved Visualization Tools
Current 3-D US imaging systems use 3-D visualization tools. However, these toolsrequire not only complicated user interfaces but also special training. In order for3-D carotid imaging to become widely accepted, we need to develop intuitive andautomated visualization tools to manipulate the 3-D image so that the user mayview the desired section of the carotid arteries using both the texture mapping andvolume rendering approaches. The production of volume-rendered images of thecarotid arteries and the acquisition of volume-rendered images of the plaque surfacerequire multiple parameters to be manipulated. Most notably, automated tech-niques are needed to provide immediate optimal rendering without significant userintervention.
Reduction in the Variability of Plaque Volume Measurement
Landry and Fenster [50] have demonstrated that the greatest source of variability inmeasurement of plaque volume (using manual planimetry) is caused by the variabil-ity of locating the initial and final slices. Outlining the plaque contributes to the vari-ability; however, this contribution is small. Figure 3.16 shows a plot of five repeatedoutlining sessions for one carotid plaque "slice" as well as the standard deviation indetermining the boundary. The mean standard deviation in the plaque outline isonly 0.28 mm. Thus, to reduce the measurement variability of the plaque volume,we need to improve our strategies for measuring the disease burden.
ECG-Triggered Acquisition of 3-D Carotid US Images
Delcker et al. [49] have shown that ECG-triggered 3-D US acquisition withfree-hand scanning resulted in improved reliability of plaque volume measurements.
However, triggered acquisition prolongs the scanning time, introduces discomfortfor the patient, and adds complexity to the instrumentation. Nonetheless, thisapproach is promising for improving the quality of the 3-D US images and reducingthe variability of plaque volume measurements.
Figure 3.16
Five contours of a plaque obtained by manual segmentation. The figure indicates
that the variability in outlining the plaque–lumen interface is small, but the variability in outliningthe plaque–vessel wall interface is higher. The greatest variability is at the lateral sides of the vessel.
The authors acknowledge the financial support of the Canadian Institutes forHealth Research and the Ontario Research and Development Challenge Fund. Thefirst author holds a Canada Research Chair and acknowledges the support of theCanada Research Chair Program.
Hegele, R. A., et al., "Infection-Susceptibility Alleles of Mannose-Binding Lectin Are Asso-ciated with Increased Carotid Plaque Area," J. Investig. Med., Vol. 48, No. 3, 2000,pp. 198–202.
Spence, J. D., M. R. Ban, and R. A. Hegele, "Lipoprotein Lipase (Lpl) Gene Variation andProgression of Carotid Artery Plaque," Stroke, Vol. 34, No. 5, 2003, pp. 1176–1180.
Spence, J. D., et al., "Carotid Plaque Area: A Tool for Targeting and Evaluating VascularPreventive Therapy," Stroke, Vol. 33, No. 12, 2002, pp. 2916–2922.
Barnett, P. A., et al., "Psychological Stress and the Progression of Carotid Artery Disease,"J. Hypertens., Vol. 15, No. 1, 1997, pp. 49–55.
Spence, J. D., et al., "Plasma Homocyst(E)Ine Concentration, But Not Mthfr Genotype, IsAssociated with Variation in Carotid Plaque Area," Stroke, Vol. 30, No. 5, 1999,pp. 969–973.
3-D US Imaging of the Carotid Arteries
[6] Hackam, D. G., J. C. Peterson, and J. D. Spence, "What Level of Plasma Homocyst(E)Ine
Should Be Treated? Effects of Vitamin Therapy on Progression of Carotid Atherosclerosis inPatients with Homocyst(E)Ine Levels above and Below 14 Umul/L," AJH, Vol. 13, 2000,pp. 105–110.
[7] Zwiebel, W. J., "Duplex Sonography of the Cerebral Arteries: Efficacy, Limitations, and
Indications," AJR Am. J. Roentgenol., Vol. 158, No. 1, 1992, pp. 29–36.
[8] Sterpetti, A. V., et al., "Ultrasonographic Features of Carotid Plaque and the Risk of Subse-
quent Neurologic Deficits," Surgery, Vol. 104, No. 4, 1988, pp. 652–660.
[9] Widder, B., et al., "Morphological Characterization of Carotid Artery Stenoses by Ultra-
sound Duplex Scanning," Ultrasound Med. Biol., Vol. 16, No. 4, 1990, pp. 349–354.
[10] Riccabona, M., et al., "In Vivo Three-Dimensional Sonographic Measurement of Organ
Volume: Validation in the Urinary Bladder," J. Ultrasound Med., Vol. 15, No. 9, 1996,pp. 627–632.
[11] Fenster, A., D. B. Downey, and H. N. Cardinal, "Three-Dimensional Ultrasound Imaging,"
Phys. Med. Biol., Vol. 46, No. 5, 2001, pp. R67–R99.
[12] Liapis, C., et al., "Internal Carotid Artery Stenosis: Rate of Progression," Eur. J. Vasc.
Endovasc. Surg., Vol. 19, No. 2, 2000, pp. 111–117.
[13] Schminke, U., et al., "Three-Dimensional Power-Mode Ultrasound for Quantification of
the Progression of Carotid Artery Atherosclerosis," J. Neurol., Vol. 247, No. 2, 2000,pp. 106–111.
[14] Serena, J., "Ultrasonography of the Progression of Atherosclerotic Plaques," Rev. Neurol.,
Vol. 29, No. 9, 1999, pp. 851–856.
[15] Ainsworth, C. D., et al., "3-D US Measurement of Change in Carotid Plaque Volume; a
Tool for Rapid Evaluation of New Therapies," Stroke, Vol. 35, 2005, pp. 1904–1909.
[16] Hatsukami, T. S., et al., "Echolucent Regions in Carotid Plaque: Preliminary Analysis Com-
paring Three-Dimensional Histologic Reconstructions to Sonographic Findings," Ultra-sound Med. Biol., Vol. 20, 1994, pp. 743–749.
[17] Steinke, W., and M. Hennerici, "Three-Dimensional Ultrasound Imaging of Carotid Artery
Plaques," J. Cardiovasc. Technol., Vol. 8, 1989, pp. 15–22.
[18] Troyer, A., et al., "Major Carotid Plaque Surface Irregularities Correlate with Neurologic
Symptoms," J. Vasc. Surg., Vol. 35, 2002, pp. 741–747.
[19] Zhao, X. Q., et al., "Effects of Prolonged Intensive Lipid-Lowering Therapy on the Charac-
teristics of Carotid Atherosclerotic Plaques In Vivo by MRI: A Case-Control Study,"Arterioscler. Thromb. Vasc. Biol., Vol. 21, No. 10, 2001, pp. 1623–1629.
[20] Yao, J., et al., "Three-Dimensional Ultrasound Study of Carotid Arteries Before and After
Endarterectomy: Analysis of Stenotic Lesions and Surgical Impact on the Vessel," Stroke,Vol. 29, No. 10, 1998, pp. 2026–2031.
[21] Sameshima, T., et al., "Clinical Usefulness of and Problems with Three-Dimensional CT
Angiography for the Evaluation of Arteriosclerotic Stenosis of the Carotid Artery: Compari-son with Conventional Angiography, MRA, and Ultrasound Sonography," Surg. Neurol.,Vol. 51, No. 3, 1999, pp. 301–308; discussion, pp. 308–309.
[22] Nelson, T. R., et al., Three-Dimensional Ultrasound, Philadelphia, PA: Lippincott-Raven,
[23] Nelson, T. R., and D. H. Pretorius, "Three-Dimensional Ultrasound Imaging," Ultrasound
Med. Biol., Vol. 24, No. 9, 1998, pp. 1243–1270.
[24] Baba, K., and D. Jurkovic, Three-Dimensional Ultrasound in Obstetrics and Gynecology,
New York: Parthenon Publishing Group, 1997.
[25] Downey, D. B., and A. Fenster, "Three-Dimensional Ultrasound: A Maturing Technology,"
Ultrasound Quarterly, Vol. 14, No. 1, 1998, pp. 25–40.
[26] Fenster, A., and D. Downey, "Three-Dimensional Ultrasound Imaging," in Handbook of
Medical Imaging, Volume 1, Physics and Psychophysics, J. Beutel, H. Kundel, and R. VanMetter, (eds.), Bellingham, WA: SPIE Press, 2000, pp. 433–509.
[27] Fenster, A., and D. Downey, "3-D US Imaging," Annual Review of Biomedical Engineer-
ing, Vol. 2, 2000, pp. 457–475.
[28] Fenster, A., and D. Downey, "Basic Principles and Applications of 3-D Ultrasound
Imaging," in An Advanced Signal Processing Handbook, S. Stergiopoulos, (ed.), BocaRaton, FL: CRC Press, 2001, pp. 14–34.
[29] Smith, W., and A. Fenster, "Statistical Analysis of Decorrelation-Based Transducer Track-
ing for Three-Dimensional Ultrasound," Med. Phys., Vol. 30, No. 7, 2003, pp. 1580–1591.
[30] Downey, D. B., and A. Fenster, "Vascular Imaging with a Three-Dimensional Power Dopp-
ler System," AJR Am. J. Roentgenol., Vol. 165, No. 3, 1995, pp. 665–658.
[31] Fenster, A., et al., "Three-Dimensional Ultrasound Imaging," Proc. SPIE Physics of Medi-
cal Imaging, Vol. 2432, 1995, pp. 176–184.
[32] Picot, P. A., et al., "Three-Dimensional Colour Doppler Imaging of the Carotid Artery,"
Proc. SPIE Image Capture, Formatting and Display, Vol. 1444, 1991, pp. 206–213.
[33] Picot, P. A., et al., "Three-Dimensional Colour Doppler Imaging," Ultrasound Med. Biol.,
Vol. 19, No. 2, 1993, pp. 95–104.
[34] Pretorius, D. H., T. R. Nelson, and J. S. Jaffe, "3-Dimensional Sonographic Analysis Based
on Color Flow Doppler and Gray Scale Image Data: A Preliminary Report," J. UltrasoundMed., Vol. 11, No. 5, 1992, pp. 225–232.
[35] Guo, Z., and A. Fenster, "Three-Dimensional Power Doppler Imaging: A Phantom Study to
Quantify Vessel Stenosis," Ultrasound Med. Biol., Vol. 22, No. 8, 1996, pp. 1059–1069.
[36] Dabrowski, W., et al., "A Real Vessel Phantom for Flow Imaging: 3-D Doppler Ultrasound
of Steady Flow," Ultrasound Med. Biol., Vol. 27, No. 1, 2001, pp. 135–141.
[37] Hughes, S. W., et al., "Volume Estimation from Multiplanar 2-D US Images Using a
Remote Electromagnetic Position and Orientation Sensor," Ultrasound Med. Biol.,Vol. 22, No. 5, 1996, pp. 561–572.
[38] Leotta, D. F., P. R. Detmer, and R. W. Martin, "Performance of a Miniature Magnetic Posi-
tion Sensor for Three-Dimensional Ultrasound Imaging," Ultrasound Med. Biol., Vol. 23,No. 4, 1997, pp. 597–609.
[39] Gilja, O. H., et al., "In Vitro Evaluation of Three-Dimensional Ultrasonography Based on
Magnetic Scanhead Tracking," Ultrasound Med. Biol., Vol. 24, No. 8, 1998,pp. 1161–1167.
[40] Barratt, D. C., et al., "Optimisation and Evaluation of an Electromagnetic Tracking Device
for High-Accuracy Three-Dimensional Ultrasound Imaging of the Carotid Arteries," Ultra-sound Med. Biol., Vol. 27, No. 7, 2001, pp. 957–968.
[41] Detmer, P. R., et al., "3-D Ultrasonic Image Feature Localization Based on Magnetic
Scanhead Tracking: In Vitro Calibration and Validation," Ultrasound Med. Biol., Vol. 20,No. 9, 1994, pp. 923–936.
[42] Hodges, T. C., et al., "Ultrasonic Three-Dimensional Reconstruction: In Vitro and In Vivo
Volume and Area Measurement," Ultrasound Med. Biol., Vol. 20, No. 8, 1994,pp. 719–729.
[43] Levoy, M., "Volume Rendering, a Hybrid Ray Tracer for Rendering Polygon and Volume
Data," IEEE Computer Graphics and Applications, Vol. 10, 1990, pp. 33–40.
[44] Allott, C. P., et al., "Volumetric Assessment of Carotid Artery Bifurcation Using Free-
hand-Acquired, Compound 3-D US," Br. J. Radiol., Vol. 72, No. 855, 1999, pp. 289–292.
[45] Delcker, A., and H. C. Diener, "Quantification of Atherosclerotic Plaques in Carotid Arter-
ies by Three-Dimensional Ultrasound," Br. J. Radiol., Vol. 67, No. 799, 1994,pp. 672–678.
[46] Delcker, A., and H. C. Diener, "3-D US Measurement of Atherosclerotic Plaque Volume in
Carotid Arteries," Bildgebung, Vol. 61, No. 2, 1994, pp. 116–121.
[47] Palombo, C., et al., "Ultrafast Three-Dimensional Ultrasound: Application to Carotid
Artery Imaging," Stroke, Vol. 29, No. 8, 1998, pp. 1631–1637.
3-D US Imaging of the Carotid Arteries
[48] Delcker, A., H. C. Diener, and H. Wilhelm, "Influence of Vascular Risk Factors for
Atherosclerotic Carotid Artery Plaque Progression," Stroke, Vol. 26, 1995, pp. 2016–2022.
[49] Delcker, A., and C. Tegeler, "Influence of ECG-Triggered Data Acquisition on Reliability
for Carotid Plaque Volume Measurements with a Magnetic Sensor Three-DimensionalUltrasound System," Ultrasound Med. Biol., Vol. 24, No. 4, 1998, pp. 601–605.
[50] Landry, A., and A. Fenster, "Theoretical and Experimental Quantification of Carotid
Plaque Volume Measurements Made by 3-D US Using Test Phantoms," Med. Phys.,Vol. 29, 2002, pp. 2319–2327.
[51] Landry, A., J. D. Spence, and A. Fenster, "Measurement of Carotid Plaque Volume by
3-Dimensional Ultrasound," Stroke, Vol. 35, No. 4, 2004, pp. 864–869.
[52] Eliasziw, M., et al., "Statistical Methodology for the Concurrent Assessment of Interrater
and Intrarater Reliability: Using Goniometric Measurements as an Example," Phys. Ther.,Vol. 74, No. 8, 1994, pp. 777–788.
[53] Mitchell, J. R., et al., "The Variability of Manual and Computer Assisted Quantification of
Multiple Sclerosis Lesion Volumes," Med. Phys., Vol. 23, No. 1, 1996, pp. 85–97.
[54] Mao, F., J. D. Gill, and A. Fenster, "Technique of Evaluation of Semi-Automatic Segmenta-
tion Methods," Proc. SPIE Image Processing, Vol. 3661, 1999, pp. 1027–1036.
[55] Mao, F., et al., "Segmentation of Carotid Artery in Ultrasound Images: Method Develop-
ment and Evaluation Technique," Med. Phys., Vol. 27, No. 8, 2000, pp. 1961–1970.
[56] Gill, J. D., et al., "Accuracy and Variability Assessment of a Semiautomatic Technique for
Segmentation of the Carotid Arteries from Three-Dimensional Ultrasound Images," Med.
Phys., Vol. 27, No. 6, 2000, pp. 1333–1342.
[57] Zahalka, A., and A. Fenster, "An Automated Segmentation Method for Three-Dimensional
Carotid Ultrasound Images," Phys. Med. Biol., Vol. 46, No. 4, 2001, pp. 1321–1342.
[58] Bluth, E. I., "Evaluation and Characterization of Carotid Plaque," Semin. Ultrasound CT
MR, Vol. 18, No. 1, 1997, pp. 57–65.
[59] Fuster, V. E. The Vulnerable Atherosclerotic Plaque: Understanding, Identification, and
Modification, Armonk, NY: Futura Publishing Company, 1999.
[60] Falk, E., P. K. Shah, and V. Fuster, "Coronary Plaque Disruption," Circulation, Vol. 92,
No. 3, 1995, pp. 657–671.
[61] Schminke, U., et al., "Three-Dimensional Ultrasound Observation of Carotid Artery Plaque
Ulceration," Stroke, Vol. 31, 2000, pp. 1651–1655.
[62] Besl, P., and R. Jain, "Invariant Surface Characteristics for 3-D Object Recognition in
Range Images," Comp. Vis. Graph. and Image Proc., Vol. 33, 1986, pp. 33–80.
[63] Han, C., T. S. Hatsukami, and C. Yuan, "Accurate Lumen Surface Roughness Measure-
ment Method in Carotid Atherosclerosis," Proc. of SPIE, Medical Imaging 2001: ImageProcessing, Vol. 4322, 2001, pp. 1817–1827.
[64] Stokeyl, E., and S. Wu, "Surface Parameterization and Curvature Measurement of Arbitrary
3-D Objects: Five Practical Methods," IEEE Trans. on Pattern Analysis and Machine Intel-ligence, Vol. 14, 1992, pp. 883–840.
[65] Barratt, D. C., et al., "Reconstruction and Quantification of the Carotid Artery Bifurcation
from 3-D US Images," IEEE Trans. on Medical Imaging, Vol. 23, No. 5, 2004, pp. 567–583.
[66] Papademetris, X., et al., "Estimation of 3-D Left Ventricular Deformation from Medical
Images Using Biomechanical Models," IEEE Trans. on Medical Imaging, Vol. 21, No. 7,2002, pp. 786–800.
[67] Besl, P., et al., "A Method for Registration of 3-D Shapes," IEEE Trans. on Pattern Analysis
and Machine Intelligence, Vol. 14, No. 2, 1992, pp. 239–256.
Source: http://www.artechhouse.co.uk/uploads/public/documents/chapters/moli-suri-144_CH03.pdf
Guidelines für kardiovaskuläre Erk rankungen in der klinischen Praxis ( ESC / AGLA ) Dr. med. Karl Nüesch Kardiologie FMH Medical manager FH/EMBA Marktstrasse 11, 9435 Heerbrugg • Vortrag über die aktuellen Richtlinien • Fallpräsentation 1 • Fallpräsentation 2 • Diskussion
Pulmonary Function Testing in ChildrenPulmonary function tests (PFT's) measure how well your child can move air in and out of his or her lungs. PFT's, also called breathing tests, will help your healthcare provider tell if your child has lung disease, how severe it is and what medications may help. Your child may be asked to do PFT's in your healthcare provider's office or in a pulmonary function laboratory (lab).