Appetite and reward
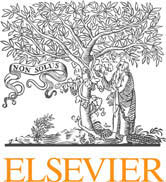
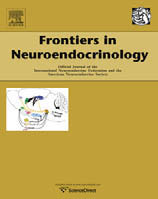
Frontiers in Neuroendocrinology 31 (2010) 85–103
Contents lists available at
Frontiers in Neuroendocrinology
Appetite and reward
Stephanie Fulton *
CRCHUM and Montreal Diabetes Research Center, Department of Nutrition, Faculty of Medicine, University of Montreal, Montreal, QC, Canada
The tendency to engage in or maintain feeding behaviour is potently influenced by the rewarding prop-
Available online 12 October 2009
erties of food. Affective and goal-directed behavioural responses for food have been assessed in responseto various physiological, pharmacological and genetic manipulations to provide much insight into the
neural mechanisms regulating motivation for food. In addition, several lines of evidence tie the actions
of metabolic signals, neuropeptides and neurotransmitters to the modulation of the reward-relevant cir-
cuitry including midbrain dopamine neurons and corticolimbic nuclei that encode emotional and cogni-
tive aspects of feeding. Along these lines, this review pulls together research describing the peripheral
Nucleus accumbens
and central signalling molecules that modulate the rewarding effects of food and the underlying neural
Ó 2009 Elsevier Inc. All rights reserved.
rience when coming into contact with an object or when engaging
‘‘We recognize pleasure as the first good innate in us, and from
in a particular behaviour can powerfully influence our approach or
pleasure we begin every act of choice and avoidance, and to
avoidance of that object or behaviour in the future. Consider how
pleasure we return again, using the feeling as the standard by
eating a delicious morsel of food propels you to take another bite.
which we judge every good." – Epicurus [Letter to Menoeceus,
Pleasurable feelings play a significant role in behaviour.
between 306 and 270 BCE]
Whether in conditions of hunger or satiety, food intake can be
influenced by the pleasurable effects of food. Thus, the joy of eatingcan arise not only from the fulfillment of a vital physiological need
but also from the sheer gratification derived from savoring appetiz-ing foods. The word pleasure often refers to a complex experience
In one of the first documented accounts of the role of pleasure
that involves emotions such as happiness, enjoyment and satisfac-
in behaviour, Epicurus captures the notion of pleasure as the expe-
tion that are difficult to evaluate in the study of non-human ani-
rience that profoundly shapes our evaluations, biases our actions
mals. Alternatively, the term reward is commonly used in the
and guides our future choices. The degree of pleasure that we expe-
scientific literature to refer to a more measurable quality of an ob-ject or action. As used here rewards (1) are objects or actions that
Abbreviations: Ach, acetylcholine; AMPA, a-amino-3-hydroxyl-5-methyl-4-
prioritize behaviour and promote the continuation of ongoing ac-
isoxazole-propionate; AMY, amygdala; ARC, arcuate nucleus; BSR, brain stimulation
tions, (2) increase the behaviours that lead to the procurement
reward; CCK, cholecystokinin; DA, dopamine; DAT, dopamine transporter; DOR,
and/or consumption of the reward (positive reinforcement), and
delta opioid receptor; DS, dorsal striatum; fMRI, functional magnetic resonanceimaging; GABA, gamma-aminobutyric acid; HIP, hippocampus; KOR, kappa opioid
(3) direct future behavioural actions.
receptor; LH, lateral hypothalamus; GP, globus pallidus; MFB, medial forebrain
Subjective estimates of the reward value of food incorporate
bundle; MOR, mu opioid receptor; mPFC, medial prefrontal cortex; NAc, nucleus
qualities such as taste, texture, smell and post-ingestive conse-
accumbens; NT, Neurotensin; OFC, orbital frontal cortex; PBN, parabrachial
quences, together with information about the amount and spatio-
nucleus; PVN, paraventricular nucleus; PI3, phosphatidylinositol-3; PYY, peptide
temporal distribution of food and the metabolic state of the
YY; NTS, nucleus of the solitary tract; NMDA, N-methyl-D-aspartic acid; 5-HT,serotonin; SN, substantia nigra; STAT3, signal transducer and activator of tran-
organism . Value representations along with information
scription; TH, tyrosine hydroxylase; VP, ventral pallidum; VTA, ventral tegmental
about the cues and properties associated with attaining food are
stored in memory to guide future behaviours, such as orient ani-
* Address: Centre de Recherche du CHUM, Technopôle Angus, 2901 Rachel E.,
mals back to the source of food. The heightened emotional and
Rm-302, Montreal, QC, Canada H1W4A4. Fax: +1 (514) 412 7648.
behavioural response to palatable high-fat and -sugar foods that
0091-3022/$ - see front matter Ó 2009 Elsevier Inc. All rights reserved.
doi:
S. Fulton / Frontiers in Neuroendocrinology 31 (2010) 85–103
has evolved can be understood in terms of what these foods offer in
obtained with electrodes along the medial forebrain bundle
caloric value. Indeed, the consumption of tasty, energy-dense foods
(MFB) and into the midbrain extension of the MFB . Self-
can produce a rewarding effect that strengthens action–outcome
stimulation is observed from electrodes located in several brain
associations and reinforces future behaviour directed at obtaining
areas, including the orbitofrontal cortex, nucleus accumbens
these foods. While the rewarding impact of eating high-fat and -su-
(NAc), lateral hypothalamus (LH), ventral tegmental area (VTA)
gar foods serves as an adaptive element in conditions of food scar-
and brainstem structures. Studies combining electrophysiological
city the increased abundance and accessibility of these foods in
linkage and immunohistochemical mapping approaches
many parts of the world has promoted excessive caloric intake
with BSR provide much insight into this multi-synaptic
and weight gain.
network of brain regions recognized for their contribution to re-
Identifying the neural circuitry and mechanisms responsible for
ward-relevant processes, including those underlying motivation
the rewarding properties of food has significant implications for
understanding energy balance and the development of obesity.
From the early findings that rats would continuously self-stim-
Although this subject matter has received widespread attention
ulate the LH while forgoing physiological needs, such as feeding
in more recent years, there is an extensive and rich body of litera-
and drinking, emerged the idea that the stimulation tapped into
ture on the study of appetite and reward. By and large, these stud-
the neural circuitry sub serving natural rewards, such as food
ies can be classified into two areas: Those investigating the impact
and water Consistent with this view, subsequent
of energy states, peptides and neurotransmitter systems on behav-
work showed that BSR establishes and maintains response patterns
ioural measures of the rewarding effects of food; and those exam-
much like those generated by natural rewards Studies of BSR
ining the modulation of reward-relevant neural circuitry by
also provided some of the first suggestions that reward circuitry is
manipulations of energy balance. To convey current understanding
subdivided along functional and anatomical lines. Rare observa-
of the behavioural processes and neural mechanisms regulating
tions of humans with self-stimulating electrodes revealed that
food reward it is important to place it in the context of our knowl-
stimulation at distinct loci could elicit different subjective reports
edge and its evolution of the neural circuitry that underlies all re-
Similarly, several lines of evidence obtained in rats provide
wards and motivated behaviours, especially since there is
strong support for anatomically segregated subpopulations of re-
considerable overlap in the associated pathways. Along these gen-
ward neurons that code for separate functions .
eral lines, this review discusses selected findings concerning: the
Investigations of the brain circuitry that gives rise to rewarding
neural basis of reward; the reward efficacy of food, and; the neural
self-stimulation not only established a neural basis for reward
pathways and mechanisms linked to food reward.
but have made great strides in characterizing the properties ofthe directly activated neurons , their connections andthe manner in which reward circuitry is functionally organized
2. Neural basis of reward
The observations that manipulations of the brain dopamine
The rewarding effects of stimuli and behaviours have long been
(DA) system modulate BSR were among the first to implicate DA
studied by measuring the willingness of the subject to work to gain
in reward-relevant processes. Certainly, the study of the neural ba-
access to the goal object. In the view of Thorndike, behavioural re-
sis of reward is well-rooted in investigations of DA circuitry. As the
sponses to stimuli that produce satisfying effects are likely to be
most studied and tied to reward-relevant functions are the meso-
repeated again . The idea that response outcomes can direct
corticolimbic and nigrostriatal DA pathways that originate in the
subsequent behavioural actions was later formulized in Skinner's
midbrain VTA and substantia nigra (SN), respectively, and project
theory of reinforcement proposing that the consequence of a re-
to various limbic and cortical sites including the NAc, amygdala
sponse acts as a positive reinforcer when the probability of that re-
(AMY), ventral pallidum (VP), dorsal striatum (DS), hippocampus
sponse occurring in the future is increased As a central tenet
(HIP) and prefrontal cortex (PFC). Early on it was shown that DA
of operant conditioning, Skinner maintained that response strength
receptor antagonists and lesioning of DA neurons via 6-
can be determined by measuring the frequency or intensity of
hydroxydopamine (6-OHDA) inhibit BSR whereas drugs that
behavioural responses (e.g. lever presses) . Operant (or
directly or indirectly increase DA tone, including amphetamine
instrumental) conditioning is a principle concept and procedure
cocaine heroine/morphine and nicotine
in the experimental analysis of behaviour and serves as an empir-
enhance the rewarding effects of MFB stimulation. Despite
ical construct in models of behavioural choice and reward mea-
the potent impact on BSR produced by modulation of DA signalling,
surement By measuring the willingness of the
the traversing of DA fibers along portions of the MFB and the facil-
subject to work for the goal object, operant measures can offer a
itatory action of MFB self-stimulation on DA release , the
meaningful index of reward effectiveness.
results of several studies suggest that the neurons directly acti-
The discovery by Olds and Milner in 1954 that rats will avidly
vated by MFB stimulation are not dopaminergic .
work to self-administer electrical stimulation to some regions of
However, the directly activated neurons may trans-synaptically
the brain was a remarkable beginning to the study of brain reward
activate DA neurons via a cholinergic input arising from the pedun-
circuitry . In addition to the eruption in the scientific commu-
culopontine or laterodorsal tegmental nucleus .
nity provoked by this finding, newspaper headlines reading the
Much like the effects of electrical brain stimulation, central
‘‘brain pleasure area" had been discovered and that ‘‘it may prove
administration of drugs that modulate DA tone can be reinforcing
the key to human behaviour" stirred up much public excitement
(see ). Several lines of evidence suggest that the reinforcing
(The Montreal Star; March 12, 1954) Also known as brain
actions of drugs of abuse are due, at least in part, to the modulation
stimulation reward (BSR), this phenomenon is considered to tap
of DA signalling in the NAc. The actions of amphetamine , co-
into the neural circuitry that conveys the rewarding properties of
caine and opiates to increase instrumental responding
natural stimuli and behaviours. The rewarding stimulation gener-
are associated with enhanced DA release in the NAc. Rats will
ates a powerful grip on behaviour as revealed by the resistance
self-administer amphetamine directly into the NAc whereas
to forgo delivery of the stimulation: Rats will cross electrified grids
selective DA lesions in the NAc block self-administration of intra-
and go without food in conditions of severe deprivation
venous amphetamine . Unlike amphetamine, rats will not
in order to maintain contact with the manipulator
learn to respond for cocaine administration into the NAc, but will
that triggers the stimulation. Robust self-stimulation behaviour is
self-administer cocaine into another DA terminal region, the
S. Fulton / Frontiers in Neuroendocrinology 31 (2010) 85–103
medial PFC (mPFC) . Nonetheless, DA release in the NAc ap-
feeding behaviour. The reinforcing effects of food have commonly
pears to be important in mediating the reinforcing actions of co-
been assessed using operant conditioning procedures that measure
caine since DA lesions in the NAc impair intravenous cocaine
the willingness to work for food. In other instances, food reinforce-
self-administration . Rats will also work for discrete injec-
ment has been studied using the conditioned place preference
tions of morphine and mu and delta opioid receptor agonists into
model. The conditioned place preference paradigm entails Pavlov-
both the VTA and NAc and injection of opiates into
ian (associative) conditioning in which the reinforcing effects of
the VTA increases extracellular levels of DA in the NAc .
the object are evaluated by measuring the amount of time the ani-
Although these data highlight the importance of NAc DA in drug
mal spends in an environment previously paired (associated) with
self-administration, a role for the mPFC in the reinforcing effects
that object Therefore, both operant conditioning and condi-
of cocaine and NMDA receptor antagonists (i.e., ketamine)
tioned place preference models measure acquired and voluntary
is well documented.
behavioural responses that are directed at obtaining the goal object
The results of experiments investigating the influence of DA on
and influenced by prior encounters with the goal object (‘‘goal-di-
BSR and drug self-administration contributed to the popular notion
rected behaviour").
that DA is responsible for rewarding behaviour. Changing dopami-
The hedonic properties of food are commonly assessed by deter-
nergic tone influences the behavioural effectiveness of rewards
mining the affective evaluations that are generated in response to
such as BSR, drugs of abuse, food and sex, however there is dis-
direct encounters with food or food-related stimuli. In the case of
agreement concerning how and where DA neurons contribute to
human participants, the respondent provides a subjective evalua-
the circuitry underlying reward. Indeed, the exact functional con-
tion of a sensory property of food (often taste) which is reflected
tribution of DA has received intense attention on both the empiri-
by a rating of pleasantness or liking. The hedonic attributes of food
cal and theoretical levels. Discrepancies in the DA literature are
have also been studied in animals using the taste reactivity para-
likely influenced by variations in DA terminal areas in the forebrain
digm which measures spontaneous oral and facial reaction pat-
under investigation differences in the electrochemical
terns to tastants that are inherent across many mammalian
techniques used and the behavioural measurements and
species . Research employing these hedonic measures of food
models employed (see
reward will be reviewed here, however relevant work addressing
Despite different views, a large body of work suggests that DA is
the role of taste, macronutrient preferences and palatability will
important for reward-relevant learning and neural plasticity. This
not be thoroughly covered. Therefore, the reader is referred to
line of research emerged from the discovery that repeated admin-
istration of drugs that stimulate DA release can have long-lasting
and palatability .
consequences by potentiating the behavioural effects of these
A commonly used measure of the reward efficacy of food is an
drugs, a process known as sensitization. Recurrent drug adminis-
operant procedure known as the progressive ratio (PR) schedule
tration has been shown to enhance locomotor-activating effects
of reinforcement. In the PR task, the subject is required to emit
an increasing number of operant responses for each successive re-
, BSR and opiate-induced feeding Notably,
ward. Eventually performance falls below a preset criterion. The
the behavioural sensitizing effects of repeated drug intake are
number of responses emitted to obtain the last reward (‘‘break
linked to elevations in DA release and long-lasting structural
point") serves as an index of the willingness to work . While
and molecular changes in DA neurons and their striatal targets
operant procedures that measure changes in response rate alone
Thus, substantial evidence has accumulated over
cannot dissociate changes in reward efficacy from alterations in
the past two decades to support a role for DA in strengthening ac-
performance capacity (e.g., ), the break point derived
tion–outcome associations; nonetheless, the precise contribution
from the PR schedule is a well-validated measure of the reward
of DA in learning-related processes and neuroadaptations is still
magnitude of food .
a matter of major investigation and discussion.
To summarize, investigations of BSR and drug self-administra-
3.1. Energy states and metabolic signals
tion have been instrumental for establishing the neural founda-tions of reward. Central to this research is the modulation of
Initial reports of PR performance for food in rats demonstrated
motivated behaviour by neurochemical manipulations revealing
that the break point declines when the caloric value of the food is
the contribution of neurotransmitter and peptide systems. Numer-
reduced whereas it increases as a function of weight loss and the
ous findings that drugs that increase DA tone can be self-adminis-
quantity of food . Both food restriction and
tered and that interfering with DA signalling in the mesolimbic
acute food deprivation increase break points and thus en-
pathway can dramatically alter BSR and drug self-administration
hance the rewarding effects of food. Break points on the PR sche-
emphasize the important role for DA in reward-relevant behaviour.
Upon discussing the impact of DA in feeding in later sections, I will
demonstrating that motivation for sucrose increases in a manner
touch on work characterizing the impact of DA on different moti-
that follows sweet taste Furthermore, food restriction and
vational and neural processes. This research deserves particular
weight loss are reported to increase sucrose motivation as a func-
attention to gain a thorough understanding of the contribution of
tion of the concentration of sucrose . These findings are com-
DA to feeding behaviour and motivation for food. As this literature
parable to those obtained in human participants that rated sucrose
will not be systematically reviewed here, the reader is referred to
solutions as more pleasant following a period of food restriction
the following reviews
and weight loss Interestingly, while the reward efficacy offood is elevated during conditions of energy deficit it is also elevated in states of increased adiposity. PR performance
3. Reward efficacy of food
for chow or sucrose is enhanced in obese Zucker rats relative tolean controls in diet-induced obese rats obesity-
Behaviour is an essential component of the energy balance
prone rats following discontinuation of a high-fat and – sugar diet
equation. Appetitive and consummatory behaviours are the sole
and in overweight as compared to lean children . These
means through which energy intake is achieved, and all behaviour
findings illustrate how PR measures can track changes in the qual-
entails energy expenditure. The rewarding properties of food can
ity of a food reward and the energy needs and motivational state of
dramatically influence the propensity to engage in or continue
the organism.
S. Fulton / Frontiers in Neuroendocrinology 31 (2010) 85–103
Human studies were the first to describe the impact of meta-
that respond to hormonal and metabolic signals from the periph-
bolic manipulations on hedonic evaluation of food. Thompson
ery to influence energy balance (this issue). Several neuropeptides
and Campbell found cellular glucopenia induced by peripheral 2-
localized in the ARC are known to regulate food intake, including
deoxyglucose (2-DG) administration increased ratings of the pleas-
the orexigenic signals neuropeptide Y (NPY), agouti-related pep-
antness of a sucrose solution . Similarly, systemic administra-
tide (AgRP) and anorexigenic signals alpha-melanocyte-stimulat-
tion of insulin was reported to enhance sucrose pleasantness
ing hormone (aMSH) and cocaine and amphetamine-regulated
ratings The process whereby insulin increases affective
transcript (CART). In addition to stimulating free-feeding intake,
ratings may be attributable to reductions in central glucose signal-
central administration of NPY potently increases the reward effi-
ling elicited by insulin given that sucrose ratings correlated nega-
cacy of chow and sucrose by increasing breakpoints in
tively with blood glucose concentrations. However, Jewett and
a PR schedule of reinforcement. NPY is also synthesized in neurons
colleagues found that glucoprivation induced by peripheral 2-DG
outside of the hypothalamus which have been linked to reward-
in rats failed to alter break points in a PR task for food while it in-
relevant processes however the actions of NPY to increase
creased the amount of freely available food consumed. The distinc-
motivation for food appears to be mediated by hypothalamic
tion between the above findings may not be simply due to species
NPY as direct administration of NPY into the perifornical LH in-
differences but rather the different measurements used, thus 2-DG
creases PR performance for sucrose . Coexpressed with NPY,
may enhance affective ratings for sweet taste without modulating
AgRP also elicits a profound increase in food intake. The actions
willingness to work measures for sucrose. Such a discrepancy be-
of AgRP to selectively increase fat appetite appears to be mediated
tween affective and instrumental measures has been described
via the MC4 receptor and mice lacking the MC4 receptor de-
and likely reflects the different neural mechanisms recruited
velop increased motivation for food in a PR task relative to wild-
Evidence obtained in rats suggests that insulin has specific ac-
type littermates . Moreover, recent evidence demonstrates
tions in the CNS to modulate the reward efficacy of food. Intraven-
that AgRP administration in rats can selectively increase the re-
tricular (ICV) administration of insulin not only decreases free-
ward efficacy of high-fat food, but not sucrose, in a PR task .
feeding intake but can inhibit PR responding for sucrose
These results provide evidence that the motivational properties
Moreover, Figlewicz et al. report that the effects of insulin to de-
of food can be divided along nutritional lines.
crease responding for sucrose is mediated via signalling in the arcu-
There is less evidence linking anorexigenic neuropeptides to the
ate nucleus (ARC) . These investigators also find that the
rewarding effects of food. Several lines of evidence link CART to
adipose-derived hormone leptin can attenuate sucrose-reinforced
mechanisms mediating the reinforcing actions of psychostimulant
responding. The above result stands in contrast to findings that ICV
drugs , yet while CART administration into the NAc de-
administration of insulin fails to alter breakpoints in a PR task for
creases breakpoints for cocaine reward it fails to alter motivation
food . The discrepancy between the two studies could be due
for food . Similarly, corticotropin-releasing hormone (CRH)
to the response measures used given that the rates of responding
decreases food intake in rats however CRH has been re-
for sucrose recorded by Figlewicz et al. may be more sensitize than
ported to increase decrease or fail to alter response
the breakpoint index measured by Jewett et al. Figlewicz et al. also
rates and the number of food reinforcers earned. In other studies,
report that insulin and leptin decrease conditioned place preference
central administration of a CRH-1 and/or CRH-2 receptor antago-
for high-fat food and thereby provide additional evidence that circu-
nists did not influence instrumental responding for food but
lating satiety hormones reflecting the status of long-term energy
a CRH-1 antagonist can attenuate the effects of stress-induced
stores can suppress the reinforcing actions of palatable foods
reinstatement of lever-pressing for palatable food Modula-
Apart from insulin and leptin, several other circulating peptides
tion of the CRH system does not appear to suppress the reward effi-
act centrally to decrease food intake. Cholecystokinin (CCK) is a
cacy of food, rather the actions of CRH to reduce food-motivated
gut-derived satiety signal that has been linked to reward-relevant
responding appear to be limited to impairments in motor capacity
neural processes Systemic CCK injection diminishes the abil-
Neurotensin (NT), on the other hand, is among the anorexi-
ity of food deprivation to increase instrumental responding for
genic signals consistently reported to inhibit instrumental
food suggesting that peripherally-derived CCK can modulate the
responding for food. NT injections into both the lateral ventricles
reinforcing effects of food . Furthermore, CCK-1 receptor defi-
and VTA decrease operant responding for food whereas
cient rats (OLETF) that are hyperphagic and obese exhibit en-
peripheral administration of a NT analog inhibits sucrose-rein-
hanced instrumental responding for sucrose relative to wildtype
forced responding . While NT is expressed in the hypothalamus
controls Released from intestinal cells in proportion to calo-
there also exist NT neuronal populations outside of the hypothala-
ric intake, peptide YY (PYY) is another gut peptide implicated in
mus with ties to feeding and reward .
appetite control In an instrumental task, peripheral admin-
Orexin-A and orexin-B are synthesized exclusively in a subset of
istration of PYY-36 failed to inhibit responding for high-fat food
LH neurons innervated by the ARC neuronal populations described
pellets, however it reduced reinstatement of food-seeking induced
above. Numerous reports over recent years demonstrate the im-
by exposure to the high-fat food and a cue associated with the food
pact of orexins on reward circuitry and its influence on goal-direc-
. Together, these data demonstrate the effects of some periph-
ted behaviour. Orexin-A infusion into the rostral LH elicits a
erally-derived satiety signals to suppress the rewarding effects of
particularly robust feeding response and enhances break-
food. There are a number of circulating peptides that have yet to
point responding for sucrose pellets In contrast, systemic
be studied in the context of food reinforcement. Specifically, it is
infusion of an orexin-1 receptor antagonist failed to alter instru-
not known whether other signals release from the digestive tract,
mental responding for sucrose . However, in another study
including ghrelin, glucagon-like peptide 1 and enterostatin, can
peripheral administration of an orexin-1 receptor blocker attenu-
modulate the reward efficacy of food. As will be reviewed later
ated instrumental responding for high-fat food pellets . Orex-
however, there is evidence tying the actions of ghrelin along with
in neurons project to the VTA and orexin-A and orexin-B
leptin, insulin and PYY to the modulation of reward circuitry.
potentiate excitatory inputs to VTA DA neurons by mechanismsinvolving both orexin-1 and -2 receptors . In addition,
3.2. Endocrine neuropeptides
Borgland et al. report that orexin-1 receptor antagonism blocks co-caine-induced locomotor sensitization and potentiation of excit-
There has been remarkable progress in the identification and
atory currents in VTA DA neurons These and other findings
characterization of the hypothalamic and hindbrain neuropeptides
implicate orexins in DA signalling and synaptic plasticity and in
S. Fulton / Frontiers in Neuroendocrinology 31 (2010) 85–103
the modulation of goal-directed behaviour for food. Like orexin,
antagonists suppress preference for sucrose and decrease
melanin-concentrating hormone (MCH) is produced exclusively
pleasantness ratings for the smell and taste of food . More-
in the LH, however in a discrete neuronal subpopulation .
over, naltrexone administration is reported to be more effective
Matching the pattern of MCH axonal projections, the MCH-1 recep-
at reducing pleasantness ratings for highly palatable foods, regard-
tor is expressed in numerous brain regions including the NAc and
less of macronutrient content . Consistent with these data,
DS . Georgescu et al. reveal a specific role for MCH signalling
morphine enhances affective responses of rats for palatable foods
in the NAc shell by demonstrating the effects on an MCH-1 recep-
as measured by the taste reactivity test Characterizing the
tor antagonist to inhibit feeding and produce anti-depressant like
receptors and brain sites mediating these opioid-induced re-
effects Consistent with these results, work of Pissios et al.
sponses, Berridge and coworker found that morphine infusion in
demonstrates enhanced behavioural responses to amphetamine
the NAc shell and DAMGO in the VP but not the LH en-
and increase stimulation-evoked DA release in the NAc shell of
hances taste reactivity patterns to sucrose.
MCH deficient mice Despite these data, it remains to be elu-
The use of instrumental response measures demonstrate that
cidated whether or not MCH enhances the reinforcing effects of
opioids can alter goal-directed behaviour for food. Peripheral injec-
tion of the non-selective opioid agonists, buprenorphine and
In summary, the data reviewed in this section illustrate that
morphine , increased break points for food on a PR schedule
several neuropeptides controlling appetite and energy balance,
of reinforcement whereas administration of naloxone produced
including NPY, AgRP, NT and orexin, can modulate goal-directed
the opposite effect . A role for MOR signalling in
behaviour for food. It is interesting that studies employing nu-
the NAc in the modulation of goal-directed behaviour for food is
clei-specific microinfusions suggest that the effects of insulin,
suggested by the demonstration that NAc DAMGO increases break-
NPY and orexin on PR performance for food are obtained with tar-
point responding for sugar pellets . MORs have a high affinity
geted injections into hypothalamic regions. This is not surprising in
for enkaphalins and endorphins and a low affinity for dynorphins
consideration of the multiple reciprocal connections between
(which are selective for kappa receptors), and both enkaphalin
hypothalamic nuclei and reward-relevant substrates. As discussed
and beta-endorphin are released in the NAc and VP. Consistent
later, leptin, insulin and ghrelin also regulate DA tone by targeting
with the above results, a series of studies by Hayward, Low and col-
midbrain DA neurons to suggest there are at least some direct ac-
leagues suggest that enkaphalin and beta-endorphin are the
tions of these hormones on reward-relevant processes.
endogenous opioid receptor ligands that mediate the actions ofopioids on the motivational properties of food. With the use of
genetically engineered mice lacking enkaphalin and beta-endor-phin these investigators report impaired breakpoint responding
The neuropeptide group most investigated in the context of the
for food in a PR task in knockouts relative to wildtype controls
rewarding effects of food and palatability are opioids, namely enk-
. Collectively, these findings highlight the important role
aphalins, dynorphins and endorphins. Each of these opioids can
of the MORs and ligands, enkaphalin and beta-endorphin, in the
influence feeding behaviour by acting on opioid receptors (mu,
NAc and VP in the rewarding effects of food and the modulation
kappa and delta) located throughout the brain. Non-selective opi-
of food palatability.
oid receptor blockade by peripheral and central naloxone administration decreases food intake in rats, especially
3.4. Neurotransmitters
when highly preferred foods such as sucrose or saccharin are used. The effect of central opioid action to modulate feed-
As with BSR and drugs of abuse, the reinforcement efficacy of
ing appears to be due, in part, to actions in the NAc and VTA. Direct
food can be modulated by alterations in DA tone. Systemic injec-
administration of opioid agonists into the NAc stimulates food in-
tions of a D1/D2 receptor antagonist can decrease instrumental re-
take whereas VTA administration of opioid agonists
sponses and conditioned place preference for food yet
or antagonists increases and decreases food intake,
fails to alter taste reactivity measures Similarly, a D2/D3
respectively. The influence of opioids in the VTA and striatum to
receptor antagonist decreases PR performance for sucrose pellets
stimulate appetite are mainly attributed to mu opioid receptors
without affecting taste reactivity patterns . The D2 but
(MOR) . The important role of MOR-induced feeding
not the D3 receptor appears to be specifically involved in food-
in the striatum is underscored by the work of Kelley and cowork-
reinforced responding given that a selective D3 receptor agonist
ers. Stimulation of MOR and delta (DOR), but not kappa (KOR)
fails to modulate PR performance and conditioned place preference
receptors in the dorsal and ventral striatum increases intake of
for food . Consistent with these findings, specific ablation of DA
chow and sucrose, while particularly robust increases in food in-
neurons in the NAc using 6-hydroxydopamine (6-OHDA) signifi-
take are seen following infusions into the NAc shell These
cantly attenuates goal-directed behaviour for food , but
investigators further show that MOR stimulation in the NAc shell
does not influence taste reactivity measures in rats and either
by D-Ala2, N-MePhe4, Gly-ol-enkephalin (DAMGO) preferentially
fails to alter or slightly augments free-feeding intake In
and dramatically increases intake of high-fat food In-
an opposite manner, Zhang et al. discovered that increasing DA sig-
creased intake of palatable foods by NAc DAMGO injection is med-
nalling in the NAc shell (and to a lesser extent the NAc core (but see
iated by the LH given that GABA receptor blockade in the LH blocks
via direct amphetamine administration increases the willing-
the feeding response . Characterizing the neural outputs
ness to work for sugar pellets on a PR schedule of reinforcement
mediating NAc MOR-induced feeding, Zheng et al. demonstrated
whereas others found that it actually inhibits free-feeding in-
that orexin neurons of the LH and their projections to the VTA
take and fails to alter taste reactivity patterns The
are involved . Apart from striatal regions, DAMGO adminis-
influence of DA on goal-directed behaviour for food is not limited
tration in the VP is also reported to increase food intake. To-
to the NAc as viral restoration of DA to the DS in DA-deficient mice
gether, these data illustrate the unique role of MOR signalling in
rescues learning and performance of an instrumental task for food
the VTA, NAc shell, DS and VP in the modulation of palatability
and feeding by opioids.
During encounters with food animals rapidly learn about the
Several lines of evidence support the idea that opioids influence
sensory qualities and the environmental cues associated with
both affective evaluations and goal-directed behaviour for food. In
attaining food. These representations are stored in memory to help
humans, systemic administration of non-selective opioid receptor
guide subsequent behavioural actions directed towards feeding.
S. Fulton / Frontiers in Neuroendocrinology 31 (2010) 85–103
The findings above illustrate how different measures provide a
of 5-HT on the reward efficacy of food Interestingly, 5-HT2C
useful way to disambiguate the influence of DA in distinct facets
receptors are located in the NAc and VTA among other loci, and 5-
of motivation for food. Collectively, the data suggest DA is impor-
HT release in the VTA from axons originating in the dorsal raphe
tant for learning about and maintaining goal-directed behaviour
inhibits DA release These findings illustrate the impact of 5-
for food rather than food consumption by itself or processes, such
HT to attenuate instrumental responding for food, an effect that
as affective reactions, that are closely connected with the consum-
may be mediated by the actions of 5-HT to reduce DA
matory phase of feeding. This view bodes well with several inves-
tigations of Salamone et al. demonstrating the impact of DA
Serving as the primary inhibitory and excitatory amino acids in
manipulations on the effort required in work-related instrumental
the brain, gamma-aminobutyric acid (GABA) and glutamate elicit
response measures . There is also general agreement in the
disparate effects on feeding depending on the site of injection
associative learning literature that DA is important to acquire
and feeding measurement employed. A GABA(A) receptor agonist
information about rewards and the behavioural responses to ob-
in the NAc shell potently increases food intake yet does not aug-
tain them. Peripheral administration of DA receptor antagonists
ment PR responding for food Correspondingly, increasing
inhibits the development of conditioned place preferences for pal-
GABAergic tone via peripheral delivery of a GABA transaminase
atable foods without affecting food consumption . In addi-
inhibitor or the GABA(B) agonist, baclofen, either modestly
tion, D1 receptor blockade in the LH and NAc shell can
decreases instrumental PR responding for food or has no effect
prevent learning of a taste aversion whereas D1 receptor blockade
. Nevertheless, in one other case systemic infusion
in the NAc core and medial PFC impairs learning of an
of baclofen significantly reduced responding for a fatty food more
instrumental task. Finally, systemic D1 receptor blockade or
so than normal chow Like GABA, glutamate signalling in
specific D1 blockade in the NAc shell or core attenuates
the NAc shell modulates food intake, an effect mediated via actions
acquisition of flavor preferences conditioned by intragastric glu-
at AMPA and kainate receptors Operant responding for
cose. Intriguingly, the results derived from studies of DA-deficient
food is reduced following peripheral AMPA antagonist (NBQX)
mice suggest that some form of learning or memory formation can
administration, but this result appears to be due to impairments
still take place in the absence of DA, but is only evident in the con-
in motor function In addition, blocking metabotropic gluta-
text of goal-directed behaviours when DA is restored to the DS
mate receptor 5 (mGluR5) by peripheral MPEP infusion did not al-
ter food-maintained responding in two reports yet
By signalling at nicotinic and muscarinic receptors, central cho-
reduced breakpoints for food in one other study . While both
linergic systems also participate in the regulation of appetite. As
GABA and glutamate plays a critical modulatory role in neuro-
the major addictive component of tobacco, nicotine acts centrally
transmission in brain regions implicated in the rewarding effects
to reduce food intake whereas smoking cessation is accompanied
of food, the data taken together do not provide strong evidence
by hyperphagia and weight gain . Nicotine administration
that these neurotransmitters by themselves have an important
into the LH suppresses food intake , an effect mediated by
influence on food-reinforced responding.
activation of nicotinic receptors located on GABA terminals that re-
Collectively, the results of behavioural studies cited above
sults in the inhibition of local MCH neurons On the other
implicate DA, Ach and 5-HT neurotransmitter systems in the mod-
hand, muscarinic receptor blockade in the SN NAc or DS
ulation of food reward. Targeted modulation of DA and Ach tone in
or selective lesion of acetylcholine (Ach) neurons in
limbic areas brings to light the influence of D1, D2 and muscarinic
the NAc potently decreases food intake. The action of musca-
receptor signalling in the NAc and dorsal striatum in the acquisi-
rinic receptor antagonists in the striatum to decrease food intake is
tion and strength of food-motivated instrumental behaviour. Sim-
associated with reductions in enkaphalin gene expression, and
ilarly, one can speculate that the effects of 5HT2C receptor
thus reduced enkaphalin binding to MORs may mediate the
stimulation to inhibit food-motivated responding could involve
anorexigenic effects Non-selective muscarinic, but not nico-
receptors expressed in the NAc. It is interesting that while these
tinic, receptor blockade in the NAc core or shell suppresses break-
neurotransmitter systems are implicated in the voluntary and
points in a PR task for sucrose and inhibits learning an
learned behavioural responses for food they do not appear ger-
instrumental task . Similarly, selective Ach lesion in the stri-
mane to affective taste reactions. Research is shedding light on
atum impairs reward-related learning . However, other inves-
the possible configuration of these pathways for the regulation of
tigators find that microinfusions of a muscarinic agonist in the NAc
food reward. Immunohistochemical localization of the various
does not increase responding for food in a PR task Despite
receptors reveals that the interaction between Ach, DA and opioid
discrepancies in the above findings, several studies implicate stri-
systems in the NAc is complex (see Clearly, more work is
atal Ach activity and release in satiety-related responses
needed in order to tease apart the neural pathways and signalling
and encoding of rewarding and aversive outcomes
events that are involved.
These actions of striatal Ach are attributed to a small pop-ulation of cholinergic interneurons that target muscarinic and nic-
3.5. Endocannabinoids
otinic receptors expressed on local striatal neurons and axonalinputs from midbrain (including DA neurons) and corticolimbic re-
The endocannabinoids, 2-arachidonoylglycerol (2-AG) and
anandamide, are lipid molecules that bind to cannabinoid (CB)
A common pharmaceutical target for weight loss treatment,
receptors to engage in numerous biological functions . Located
serotonin (5-HT) has long been known to influence appetite. By
on presynaptic terminals releasing GABA or glutamate, the CB1
means of pharmacological and genetic tools, 5-HT1B, 5-HT2C and
receptor is widely expressed in the brain, including moderate to
5-HT6 subtypes were shown to be the primary receptors through
strong levels of expression in cortex, HIP, AMY, striatum and
which 5-HT exerts its anorectic effects . Chronic blockade or
HYP. Due to its localization on inhibitory and excitatory presynap-
deletion of the 5-HT transporter decreases instrumental respond-
tic terminals, retrograde activation of CB1 receptors can regulate
ing for food . Direct infusion of 5-HT into the NAc inhibits
the release and/or signalling of several neurotransmitters and neu-
operant responding for food in a PR task, an effect that is not med-
ropeptides, including dopamine, MCH and orexin and are thus con-
iated by the 5-HT1B receptor However, peripheral infusion
of a selective 5-HT2C agonist is reported to decrease PR responding
The endocannabinoid system has received much recent atten-
for food suggesting a role for this receptor in mediating the action
tion for its role in regulating food intake and palatability. As the ac-
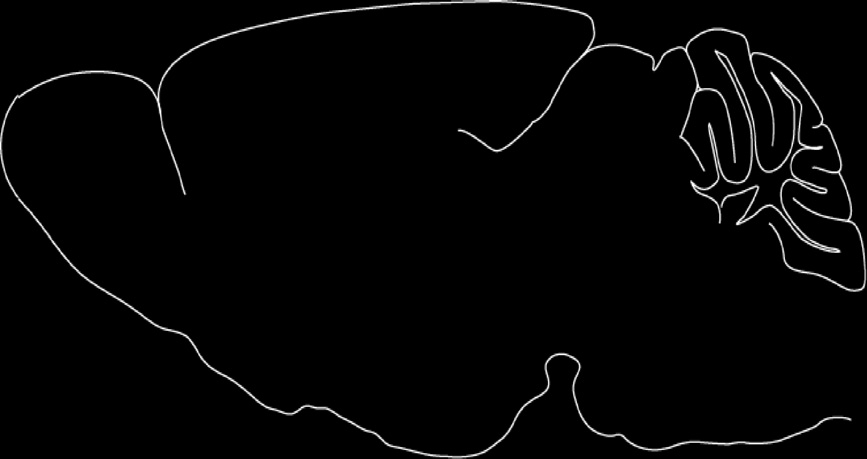
S. Fulton / Frontiers in Neuroendocrinology 31 (2010) 85–103
Fig. 1. Model of basal ganglia circuit organization This prominent model consists of parallel ascending cortico-basal ganglia–thalamus–cortico ‘‘loops". The NAcsends projections back to the source of DA neurons in the midbrain VTA. VTA DA neurons that receive afferents from the NAc shell send DA projections back to the NAc shelland core. In turn, NAc core neurons project back to the DA neurons from which they received input in addition to DA neurons of the SN that innervate the DS . Thismodel has been used to explain the process whereby goal-directed behaviour persists and can eventually develop into a habit by a gradual shift in its control from ventralstriatal regions to more dorsal areas of the striatum .
be obtained with targeted injections in the NAc shell region
Moreover, anandamide injections in the NAc shell significantly in-crease affective taste reactivity patterns for a sucrose solution
There is growing evidence for an interaction between endo-cannabinoids and opioids in the regulation of food preferences andfood reward. Solinas and Goldberg discovered that the effect ofTHC to increase breakpoints for food in a PR task could be blockedby the non-specific opioid antagonist naloxone . In turn, these
authors show that the effect of morphine to enhance breakpoints is
prevented by administration of the CB1 antagonist rimonabant.
Intriguingly, CB1 receptors are co-localized with MORs on the pro-
cesses of striatal medium spiny neurons and there is evi-
dence for functional interaction between these receptors in the
NAc that regulates the release of GABA and glutamate Tosummarize, endocannabinoids in the NAc shell enhance the
Fig. 2. Striatal targets of midbrain DA neurons and their outputs. GABAergic
rewarding effects of food, possibly via a CB1 receptor – MOR inter-
medium spiny neurons exist in two subpopulations: (1) GABA/dynorphin neurons
action. CB1 receptors are also present in the VTA where they in-
that mainly express D1 receptors and project directly to basal ganglia output nuclei
crease DA firing and release and thus endocannabinoid
including the SN (striatonigral pathway), (2) GABA/enkaphalin neurons that expresshigh levels of D2 receptors and MORs and project indirectly to basal ganglia output
action in the VTA may contribute to the modulation of food reward.
nuclei (striatopallidal pathway). Only the signalling molecules and receptors(denoted by symbols) implicated in the rewarding effects of food are illustrated.
Ach, acetylcholine; CB1, cannabinoid-1 receptor; D1; dopamine-1 receptor; D2,dopamine-2 receptor; Dyn, dynorphin; Enk, enkaphalin; M, muscarinic receptor(mostly M1 and M4); MOR, mu opioid receptor. Illustration created thanks to
A great deal has been discovered about the signals and neural
Servier Medical Art.
pathways contributing to the rewarding effects of food by investi-gations of the neurochemical and genetic basis of affective andgoal-directed behaviour for food. The NAc shell stands out as a
tive ingredient in cannabis, delta-9 tetrahydrocannabinol (THC),
key brain region that coordinates neurotransmitter, opioid and
has long been known to increase food intake, particularly for palat-
endocannabinoids signals to control feeding behaviour. Among
able sucrose Moreover, THC enhances the rewarding effect
the important signalling molecules in the NAc shell are DA, Ach,
of food by increasing PR responding for food pellets A
opioids (enkaphalin and beta-endorphin) and cannabinoids and
specific role for the CB1 receptor in the reinforcing actions of food
their respective actions at DA (D1 and D2), muscarinic (likely M1
is highlighted by several demonstrations that CB1 antagonism de-
and M4), MOR and CB1 receptors. The means by which these differ-
creases instrumental responding for foods .
ent signals interact to differentially modulate free-feeding intake,
These data are supported by finding obtained in CB1 receptor
food-motivated behaviour versus affective reactions for food re-
knockout mice demonstrating reduced responding for sucrose
mains to be fully elucidated. For instance, MOR and CB1 receptor
. However, when standard food pellets served as the rein-
activation in the NAc shell enhances goal-directed and affective re-
forcer, CB1 receptor knockout mice were not different than controls
sponses for palatable foods in addition to free-feeding intake. In
. Indeed, the effects of a CB1 receptor antagonist or agonist to
contrast, increasing DA signalling in the NAc strengthens instru-
decrease and increase, respectively, food-reinforced responding are
mental behaviour yet fails to augment free-feeding intake and
reported to be selective for a sweet reinforcer as compared to a
taste reactivity responses. Finally, while GABA A receptor activa-
pure fat reinforcer
tion in the NAc shell stimulates free-feeding it fails to augment
Much like opioid-induced feeding, a particularly robust stimula-
PR responding for food. The distinction between the behavioural
tory effect of the endogenous CB1 receptor ligand, anandamide, can
responses produced by these signals undoubtedly lies in the spe-
S. Fulton / Frontiers in Neuroendocrinology 31 (2010) 85–103
cific neuronal subpopulations and outputs involved. Research has
one subpopulation consists of GABA/dynorphin neurons that
made progress in delineating the anatomical and physiological
mainly express D1 receptors and project directly to basal ganglia
interactions between neurotransmitter and peptide systems in
output nuclei including the SN, also known as the direct or stria-
midbrain and corticolimbic areas. The findings from these studies,
tonigral pathway. The other pathway consists of GABA/enkapha-
which will be elaborated on in the next section, can provide valu-
lin neurons that express high levels of D2 receptors and MORs
able input for dissecting the processes underlying different facets
and project indirectly to basal ganglia output nuclei via the exter-
of food reward. As this circuitry is uncovered we will be able to
nal GP and subthalamic nucleus, also known as the indirect or
gain more insight into the genes and epigenetic modifications that
striatopallidal pathway. Integration between these parallel striatal
promote individual susceptibility to intake of rewarding high-fat
outputs and their corresponding circuit loops (as described
and sugar foods as a means to determine which mechanisms are
above) is considered to be essential for coherent behaviour
contributing to overeating and obesity.
and Ach interneurons are purported to play a role in thecommunication between the different pathways and striatal com-partments . Each striatal output pathway is differen-
4. Neural pathways and mechanisms of food reward
tially modulated by DA: DA excites the direct pathway via theD1 receptor whereas it inhibits the indirect pathway by way of
4.1. Corticolimbic circuitry: basic anatomy and function
the D2 receptor . In this regards, it is interesting that themajority of NAc neurons differentially respond to either primary
The central nervous system integrates numerous metabolic sig-
rewarding or aversive taste stimuli and to the cues that predict
nals from the periphery and thereby generates adaptive behav-
of their availability. Specifically, some NAc neurons are inhibited
ioural responses to changing energy demands. Information about
by tasting a sucrose solution (or exposure to a cue paired with su-
the metabolic state of the organism is preferentially encoded in
crose) whereas others are excited by an aversive quinine solution
hypothalamic and hindbrain sites whereas emotional and cogni-
, and these actions are opposite to the effects of these
tive aspects are processed in limbic and cortical loci that assign sal-
tastants on DA neurotransmission in the NAc shell While
ience and motivational valence to objects and behavioural actions.
these findings demonstrate anatomically and functionally distinct
As used here, corticolimbic substrates refer to components of cor-
NAc outputs in response to rewarding versus aversive taste stim-
tex, striatum and pallidum which each give rise to descending pro-
uli it should be noted that it is not known whether these differ-
jections to the motor system (including basal ganglia output nuclei)
ences reflect direct and indirect striatal output pathways.
whereby motivation is converted into action This circuitry is
The NAc is considered as an interface for emotion, motivation
organized such that ventral components, including the AMY and
and action due to its excitatory glutamatergic inputs from limbic
NAc, are more implicated in the processing of emotions and emo-
and cortical structures such as the AMY, HIP and PFC .
tional learning, and more dorsal portions, including the PFC and DS,
Among the limbic inputs to the NAc is the AMY which receives
are more involved in cognitive processes and habit formation
food-related sensory information from the hindbrain and the cor-
tex, in addition to physiological signals related to hunger and sati-
The neural networks that give rise to goal-directed behaviour
ety via hindbrain nuclei . Moreover, the AMY is a key
rely on basal ganglia outputs to channel motivation into behav-
substrate for processing emotion and associative condition-
ioural action. Central to many research and theoretical approaches
ing of food-related instrumental responses . These and other
is an influential model of basal ganglia circuit organization which
observations suggest that the AMY connects external and internal
consists of parallel ascending cortico-basal ganglia–thalamus–cor-
sensory information with motivational systems of the brain. Pro-
tico ‘‘loops" As a component of one circuit, the
viding additional input to NAc, the HIP serves a crucial role in
NAc sends projections back to the source of DA neurons in the mid-
memory formation and retrieval of internal and external cues,
brain VTA. VTA DA neurons that receive afferents from the NAc
and new developments link the HIP to the control of food intake
shell send DA projections back to the NAc shell and core. In turn,
Finally, the PFC is responsible for higher-order cognitive
NAc core neurons project back to the DA neurons from which they
processes related to attention, working memory, decision-making
received input in addition to DA neurons of the SN that innervate
and planning . The mPFC receives input from insular cortex
the DS Although often linked with regulation of motor
regions that relay gustatory information , and mPFC inputs
control, the DS and its DA inputs from the SN are well-implicated
to the NAc have an important influence on NAc signalling
in reward-relevant learning and habit formation ). This
. As a common element among the NAc, AMY, HIP and
model serves as an intriguing conceptual framework to describe
PFC are the inputs of DA neurons originating in the VTA that to-
the process whereby goal-directed behaviour becomes less flexible
gether form the mesocorticolimbic DA pathway ().
and more persistent (as during the development of addiction) by a
The anatomical and functional division of the NAc into different
gradual shift in its control from ventral striatal regions to more
subterritories, namely the shell and core, is well-recognized .
dorsal areas of the striatum While the relevancy of this model
Pharmacological manipulation of opioid, cannabinoid, GABA, gluta-
to the behavioural controls of feeding remain to be empirically
mate and MCH receptor signalling selectively in the NAc shell pro-
tested, it is interesting to consider how such processes may partic-
duces particularly robust effects on feeding which is
ipate in motivation and craving for palatable high-energy foods
reflected by the convergence of neurotransmitter and peptide sig-
and overeating.
nals in this region. Consistent with its distinctive role in the regu-
The striatum receives the bulk of DA afferents from the mid-
lation of feeding behaviour and food reward, the NAc shell is
brain. The majority of striatal neurons are medium spiny neurons
innervated by subcortical structures involved in energy homeosta-
that are so-called because of their high spine density ().
sis including the ARC, LH, VTA, nucleus of the solitary tract (NTS)
There is also a small subset of cholinergic interneurons localized
and parabrachial nucleus (PBN) . In turn, the NAc shell sends
to the striatum with dense local arborizations that are
descending outputs to feeding-related sites such as the VP, VTA
implicated in satiety-related mechanisms sucrose
bingeing and in the encoding of rewarding and aversive out-
With its reciprocal connections with the NAc, the LH serves as
comes . Medium spiny neurons express GABA and can be
an important moderator between limbic motivational processes
grouped into at least two different subtypes based on their axonal
and motor output pathways (). A long line of neurophysiologi-
projections and gene expression . As illustrated in
cal and neuroanatomical research implicates the LH in the
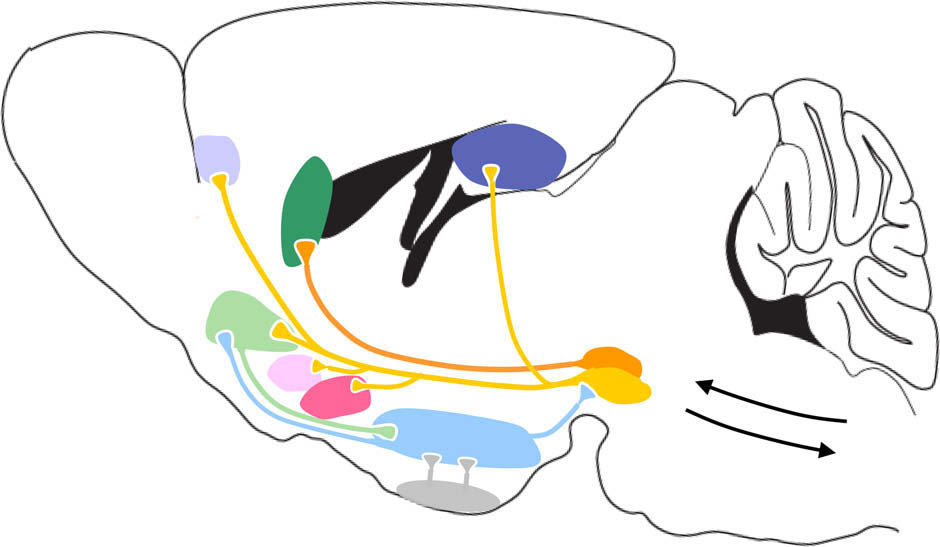
S. Fulton / Frontiers in Neuroendocrinology 31 (2010) 85–103
Fig. 3. Corticolimbic circuitry integrates metabolic information via multiple inputs. Present knowledge of the sites targeted by leptin, insulin and ghrelin to impact reward-relevant behaviour and circuitry. Leptin targets the VTA and LH neurons that project to the VTA , inhibits food intake when infused into these nucleiand is important for regulating DA tone Insulin targets the VTA and striatum and reduces DA tone VTA insulin decreases MOR-inducedfeeding while ARC insulin inhibits sucrose responding Ghrelin targets VTA DA neurons , enhances DA tone and increases food intake whenadministered to the VTA and NAc Midbrain DA neurons project to limbic and cortical sites that regulate emotion, cognition and learning. The NAc is well-positionedto assimilate information about emotion, cognition, metabolic state and gustation arising from AMY, mPFC and HIPPO, ARC, LH, VTA and hindbrain afferent inputs (not alldepicted here). Corticolimbic nuclei send descending projections to basal ganglia motor outputs to convert motivation into behavioural actions. GABAergic medium spinyneurons of the NAc shell (feeding hotspot) also reach motor output pathways by way of LH neurons that are critical for NAc shell modulation of feeding behaviour.
regulation of energy homeostasis. LH cells can respond to the taste
will be discussed in the next section, studies of brain stimulation
, sight and smell of food and receive gustatory
reward (BSR) implicate a subset of reward-related neurons located
afferents from the thalamus and hindbrain . Moreover,
in or coursing through the perifornical LH in the regulation of en-
LH cells receive information about peripheral energy stores from
ergy balance.
hormones like leptin nutrients signals such as glu-cose and afferents originating from energy-sensing mecha-
4.2. Brain stimulation reward and energy balance
nisms in the ARC
Among the important LH neuronal populations involved in the
The landmark study of Anand and Brobeck in 1951 showing that
control of food intake are those expressing the orexigenic peptides
bilateral electrolytic lesions of the LH resulted in a profound de-
orexin and MCH. MCH and orexin neurons innervate several re-
crease in feeding, drinking and body weight likely inspired the
gions including the NAc and VTA, respectively, and thus may estab-
view that the LH signals the rewarding properties of foods
lish another means whereby information about metabolic state
Thereafter, similar findings documented the reliable symptomolo-
and sensory modalities influence corticolimbic circuitry. Corre-
gy of LH electrolytic lesions which collectively became known as
spondingly, increased food intake by MOR or GABA receptor
the LH syndrome. The significance of the LH was reinforced by
stimulation in the NAc shell appears to rely on projections be-
demonstrations that electrical stimulation through the same LH
tween the NAc and the LH that stimulate orexin neurons. More-
electrode that could give rise to the BSR could also elicit a feeding
over, orexin-1 receptor signalling in the VTA is an essential
response Furthermore, using conventional rate measures,
downstream mediator of the process whereby MOR stimulation
self-stimulation of the LH in rats was shown to increase in re-
in the NAc shell enhances high-fat feeding . Thus, LH orexin
sponse to acute food deprivation and decrease following
neurons may be part of a striatal–hypothalamic–basal ganglia–
force feeding and spontaneous meal consumption in a manner
striatal loop which controls intake of fatty foods. Finally, recent
resembling the influence of these manipulations on food intake
findings demonstrate the existence of a subpopulation of GABAer-
Collectively, these findings encouraged the notion of
gic LH neurons that express leptin receptors and project to the VTA.
the LH as a ‘‘feeding center" (see
Direct leptin administration into the LH decreases food intake
Subsequent studies employing threshold measures (e.g., curve-
while increasing VTA TH expression and NAc DA content in ob/ob
shift method ) to determine changes in the reward effective-
leptin-deficient mice that exhibit diminished TH expression in
ness of BSR yielded different results. An acute period of food depri-
the VTA. Still much remains to be learned about the LH and the dis-
vation has little or no effect on rate-frequency thresholds for BSR
tinct connections and characteristics of its subdivisions .
In addition, while rats choose between gustatory stimuli
The complexity of this heterogeneous area is also derived from the
and the LH stimulation in a manner that implies that the two re-
numerous fibers of passage coursing rostrally and caudally through
wards are being evaluated along a common dimension, there is
more lateral portions of the LH that comprise the medial forebrain
compelling data that self-stimulation can be quite different from
bundle (MFB) . The MFB is a common locus for electrical stim-
the signal generated by a rewarding piece of food When
ulation that gives rise to rewarding self-stimulation behaviour. As
rats are given a choice between LH stimulation and either a sucrose
S. Fulton / Frontiers in Neuroendocrinology 31 (2010) 85–103
reward alone or a compound reward consisting of sucrose plus a
scribed by Shizgal et al., one possibility is that the restriction-sen-
fixed train of stimulation, Conover and Shizgal found that BSR re-
sitive subpopulation of reward neurons is tied to the regulation of
mained stable as the sucrose solution accumulated in the gut
non-ingestive behaviours that defend body weight, such as food
whereas responding for the compound reward was substantially
hoarding Indeed, food hoarding behaviour is highly corre-
suppressed . Therefore, models that proposed that rewarding
lated with body weight, as rats loose weight they will hoard pro-
LH stimulation is analogous to food reward are faced with para-
portionally more food . Moreover, food hoarding is not
doxical findings that manipulations that dramatically increase food
influenced by acute food deprivation nor NPY administration
intake largely fail to alter BSR in a similar manner.
An alternative possibility draws on developments regard-
There are several lines of evidence suggesting that stimulation
ing the circuits and peptides affecting fat intake to suggest that
of a subset of LH sites close to the fornix activates a stage of reward
the restriction-sensitive subset of reward neurons contribute to
circuitry that contributes to the regulation of energy balance. Early
the rewarding properties of high-fat food. Consistent with this
investigations of Blundell and Herberg showed that response rates
view is evidence that preference for high-fat food is modulated
for rewarding LH stimulation increased with chronic food restric-
by manipulations similar to those affecting BSR at restriction-sen-
tion and body weight loss only when the stimulating electrodes
sitive sites, including chronic food restriction and weight loss
were located in the perifornical region of the LH Carr and
and opioids . These ideas remain speculative but provide
Wolinsky obtained similar results when employing thresholds to
interesting prospects for empirical investigation.
measure changes in BSR over a period of food restriction andweight loss. In the same study these authors show that ICV injec-
4.3. Dopaminergic correlates of feeding
tion of the non-selective opioid antagonist, naltrexone, reversesthe potentiation of BSR by food restriction at perifornical sites
Based on his classical studies in the early 1970s in which severe
. The modulation of BSR by weight loss has been replicated
aphagia and adipsia was induced by 6-OHDA DA lesions, Unger-
several times , and depends on the site of stim-
stedt proposed that the LH syndrome resulting from lesions of
ulation in the LH . These data suggest that stimulation of the
the LH is the consequence of damage to ascending DA pathways
LH recruits at least two anatomically and functionally distinct sub-
Later work demonstrated that the feeding and metabolic
populations of rewards neurons, one of which is linked to the reg-
impairments could result from the loss of intrinsic LH neurons
ulation of body weight and can be activated by stimulating
(see ), nonetheless, this early demonstration was the beginning
neurons residing in or coursing through the perifornical LH.
of an extensive line of work investigating the impact of DA in feed-
Through which circulating signals does food restriction and
ing. Indeed, a central focus of much of the research on the neuro-
weight loss enhance the rewarding effect of perifornical stimula-
chemical basis of feeding is the mesocorticolimbic DA pathway.
tion? Fulton et al. investigated the impact of ICV leptin on the
To set the stage for evidence that DA neurons and their inputs
rewarding stimulation obtained from LH self-stimulation sites that
are targets of hormones involved in energy balance, key findings
are either sensitive or insensitive to weight loss Leptin sup-
regarding the physiological properties of DA neurons and their re-
pressed the rewarding effect of the stimulation only at ‘‘restriction-
sponses to feeding are discussed below.
sensitive" perifornical sites, thus leptin reversed the effects of
There has been much research devoted to understanding the
weight loss on BSR. Conversely, leptin enhanced the rewarding ef-
regulation of firing patterns of DA neurons and several influential
fect of the stimulation at the majority of ‘‘restriction-insensitive"
findings have tied the activity state of DA neurons to specific cod-
sites. These contrasting effects of leptin at restriction-sensitive
ing and behavioural functions. DA neurons recorded in vivo are re-
and -insensitive sites are interpreted in terms of the different ways
ported to exhibit three patterns of activity: an inactive state; a
in which leptin can contribute to the regulation of energy balance
slow, single-spike state, known as tonic firing pattern; and a burst
On the one hand, leptin may suppress the reward-
or phasic mode The tonic firing pattern of DA neurons re-
ing effects of behaviours that promote energy intake while, on
sults in relatively low and more diffuse extracellular DA levels
the other, augment those compatible with increased energy expen-
and is driven by an intrinsic pacemaker , whereas phasic
diture, such as physical activity. Along with leptin, the circulating
activity has been show to rely on afferent input to DA neurons
levels of insulin also vary as a function of the amount of adipose
and to produce high synaptic DA concentrations which acti-
tissue. Two reports by Carr and colleagues implicate insulin in
vate post-synaptic DA receptors . Importantly, the phasic
the modulation of BSR by food restriction and weight loss. In one
pattern of DA firing and release has been functionally tied to goal-
study they found that streptozotocin administration, a manipula-
directed behaviour and the prediction of rewards . The
tion that decreases insulin levels, potentiated the rewarding effects
dynamics of DA release in mesolimbic and nigrostriatal DA path-
of the stimulation . Conversely, ICV administration of insulin
ways are also influenced by D2-autoreceptors which provide neg-
attenuates the rewarding effect of the stimulation Leptin
ative feedback by rapidly inhibiting DA release . Bearing in
and insulin may alter BSR via their well-characterized influence
mind these and other characteristics, it is increasingly evident that
on neuropeptide systems. However, while central CRH and NPY
striatal DA transmission is not a unitary phenomenon, but can be
infusions altered food intake they largely failed to modulate BSR
separated into distinct functional components based on DA termi-
at restriction-sensitive sites Together, the data suggest
nal region and activity state.
that CRH and NPY are not intermediates in the process whereby
DA transmission in the NAc has been linked exclusively with
leptin and insulin modulate the rewarding stimulation at restric-
the consummatory rather than anticipatory aspects of feeding
tion-sensitive sites, but do not exclude the possibility that other
(e.g., and related to the amount of food ingested .
neuropeptides, such as AgRP and orexin, could be involved.
Nonetheless, the results of several studies suggest that DA firing
Collectively, the data demonstrate that food restriction, opioids,
and release is closely tied to novel food stimuli and/or to the re-
leptin and insulin alter a subset of reward-relevant circuitry in a
sponses and stimuli that are predictive of food reward. Using
manner that is consistent with their impact on behaviours that
in vivo microdialysis to sample extracellular DA concentration,
contribute to energy intake. However, it is not plausible that
early studies of Hoebel and colleague found DA levels are elevated
restriction-sensitive reward neurons produce a signal of that is
in the NAc during lever-pressing for food in food-restricted rats
generally related to hunger given that manipulations that increase
and DA release remains elevated during and after food consump-
the rewarding effects of food, including acute food deprivation, do
tion . In other studies, DA release in the NAc is specifically
not modulate BSR at restriction-sensitive sites As de-
associated with the instrumental response required to get access
S. Fulton / Frontiers in Neuroendocrinology 31 (2010) 85–103
to familiar food but not with food consumption an effect that
DA is critical for long-lasting cellular changes in the striatum,
is more pronounced in the NAc shell region Bassareo and Di
including homeostatic neuroadaptations synaptic plasticity
Chiara found that DA release is stimulated in the NAc shell during
and structural modifications . In particular, there
initial exposure and consumption of novel food but not during sub-
have been great advancements in our understanding of the molec-
sequent presentations, suggesting that DA release undergoes
ular mechanisms in the NAc that correspond to behavioural adap-
habituation to food stimuli . These authors also find that DA
tations in response to rewarding drugs of abuse in which DA is
is preferentially released into the NAc shell as compared to the core
purported to play a central role . How these molecular adap-
in response to unpredicted food, but following repeated exposure
tations figure in the regulation of food reward is beginning to be
to food stimuli the DA response shows habituation only in the shell
In a compatible manner, DA release is stimulated in the NAcshell only during conditions in which a food reinforcement is
4.4. Impact of metabolic signals on reward circuitry
unpredictable . Similar results have been obtained with singleunit recordings whereby VTA DA neurons exhibit phasic firing in
Of the objectives that must be met for an animal to survive the
response to unpredicted food rewards . However, once food
goal of maintaining adequate energy levels occupies a command-
is associated with stimuli that predict its availability, phasic DA
ing position. The energy state of the animal not only influences
activation is then triggered in response to these predictive stimuli
behaviours oriented towards attaining and consuming food but
also has direct bearing on all other behavioural actions necessitat-
Employing cyclic voltammetry which measures DA release over
ing energy expenditure. Thus, it is not surprising that the brain
much shorter-time frames (100 ms) than microdialysis, Roitman
comprises numerous mechanisms by which it can sense the status
et al. discovered that phasic DA released is stimulated in the NAc
of energy fuels so that it may adjust sensory, autonomic and
core in response to familiar sucrose-associated cues and peaks dur-
behavioural systems to efficiently meet energy demands. The
ing lever-pressing responses for sucrose reward but returns to
study of the pathways and mechanisms by which hormones and
baseline during food consumption In another study that uses
nutrient signals influence food intake has substantially advanced
novel food stimuli, these investigators found that phasic DA release
our knowledge of the CNS controls of energy homeostasis. The
is triggered in the NAc shell during oral sucrose infusions which
view that sensing of peripherally-derived energy signals is the
persisted during the consummatory phase In contrast, these
exclusive function of hypothalamic and hindbrain cells has been
authors report that intra-oral infusions of novel aversive stimuli
modified by several lines of evidence indicating that hormonal sig-
(quinine) produced significant decreases in DA release. In a reverse
nals, like leptin and insulin, target neuronal populations through-
manner, a subset of NAc neurons are inhibited by tasting a sucrose
out the brain to affect sensory modalities,
solution whereas another subset are excited by quinine to suggest
biological rhythms, memory and reward-relevant processes.
that behavioural responses to rewarding and aversive stimuli are
The modulation of reward circuitry by leptin has been described
anatomically divided at the level of the NAc and its outputs
in both rodents and humans. Leptin inhibits the rewarding effects
. Interestingly, NAc DA release and the activity of NAc neu-
of LH self-stimulation goal-directed behaviour for food
rons in response to sucrose ingestion are not contingent on func-
food-deprivation induced heroin seeking and en-
tional taste transduction suggesting that DA release can be
modulated by post-ingestive controls that condition feeding
Shedding light on the sites that may mediate such ef-
fects, leptin receptors are localized to midbrain DA neurons
DA release has been measured during conditions of feeding in
and leptin administration decreases basal and feeding-
DA terminal regions other than the NAc, although these reports
evoked extracellular DA levels in the NAc shell Demonstrat-
are less common. DA release is elevated following feeding in the
ing a direct action of leptin on DA neurons, Hommel et al. report
DS but not the HIP in food deprived rats . In contrast, another
that infusion of leptin in the VTA activates STAT3 in DA neurons
study found no increase in extracellular DA release in the DS dur-
and decreases food intake Conversely, conditional leptin
ing and following food intake Consumption of a novel food
receptor knockdown in the VTA increased food intake and locomo-
stimulates DA release in the mPFC in non-deprived rats and
tor activity. As a potential mechanism mediating the influence of
mPFC DA release has been shown to increase just prior to the deliv-
leptin on feeding, these authors demonstrate that systemic leptin
ery of predictable and familiar food rewards but not during food
administration or direct leptin application to the slice bath de-
consumption . Moreover, mPFC DA release has been shown
creases the tonic firing of VTA DA neurons. Complementing these
to exert an important inhibitory influence on NAc DA release and
findings, Fulton et al. reported that leptin activates STAT3 in DA
food-reinforced responding . Finally, there is a strong correla-
and GABA neurons of the VTA and that a subset of pSTAT3-positive
tion between the magnitude of DA release in the mPFC and perfor-
neurons project to the NAc core and/or shell Obese leptin-
mance on a food-associated memory task
deficient ob/ob mice showed substantially reduced locomotor re-
As illustrated by the studies above, there are different DA re-
sponses to amphetamine and failed to sensitize to repeated
sponses in the NAc shell versus core which reflect the functional
amphetamine administration, impairments which were restored
differences between these compartments and their inputs .
by peripheral leptin infusion . As an explanation for the
DA release is elevated in the NAc and mPFC during both appetitive
diminished locomotor response to amphetamine, stimulation-
and consummatory phases of feeding for novel food, but phasic DA
evoked DA release in the NAc shell was significantly reduced in
release in the NAc shell is no longer triggered during food con-
ob/ob mice along with DA and TH content in the NAc. This finding
sumption upon subsequent presentation of the food. Thus, in the
is in agreement with evidence of Roseberry et al., showing that co-
case of familiar foods, DA release in the NAc and mPFC appears
caine-induced somatodendritic DA release, as measured by D2
to be preferentially linked to predictive instrumental responses
autoreceptor inhibitory post-synaptic potentials, is reduced in ob/
or conditioned stimuli rather than actual food consumption. DA re-
ob mice . These authors also provide evidence to suggest that
lease in the mPFC appears to also play a role in the retrieval of cue-
impaired vesicular packaging of DA contributes to reduced DA re-
associated memories that correctly guide animals towards food.
lease in ob/ob mice. Finally, Leinninger et al. recently demonstrated
These finding are consistent with the view that DA serves as a
that infusions of leptin into the LH reduce food intake and increase
teaching signal to influence future commerce with food stimuli.
VTA TH and NAc DA content in ob/ob mice. These investigators
Consistent with these data is the wealth of evidence showing that
identify a novel subpopulation of GABAergic LH neurons that
S. Fulton / Frontiers in Neuroendocrinology 31 (2010) 85–103
innervate the VTA and are functionally targeted by leptin, and
the VTA or NAc increases food intake in rats This orexigenic
thereby reveal a means by which leptin may modulate DA avail-
effect of ghrelin may be due to its ability to stimulate DA release
ability in the mesoaccumbens pathway.
as central ghrelin administration potentiates extracellular levels
Human imaging research draws attention to the NAc as a locus
of DA in the NAc . Illustrating the direct action of ghrelin
mediating the impact of leptin on food reward. Farooqi et al. dem-
on VTA DA neurons, Abizaid and coworkers demonstrated that
onstrated that NAc activation, as measured by functional magnetic
ghrelin binds to VTA cells and increases DA firing The in-
resonance imaging (fMRI), is positively correlated with affective
crease in DA firing is associated with ghrelin-induced increases
ratings of visual food stimuli in fed and fasted leptin-deficient hu-
in the number excitatory synaptic inputs and decreased inhibi-
mans . Following leptin treatment the association between
tory inputs to VTA DA neurons observed by these authors. These
NAc responses and affective reactions remain in the fasted condi-
investigators also find that ghrelin increases DA turnover in the
tions but disappear in the fed state. These findings do not implicate
ventral striatum and stimulates food intake when administered
changes in leptin signalling to altered DA tone in the NAc, however
into the VTA. Likewise, NAc DA release is elevated following di-
they pinpoint the NAc as a site that integrates information about
rect VTA infusion of ghrelin whereas recent work suggests
leptin signalling, metabolic state and affective responses for food.
that peripheral ghrelin stimulates DA release exclusively in the
Collectively, the rodent and human studies demonstrate that leptin
NAc shell and not the core . Finally, ghrelin is reported to
modulates feeding behaviour via direct actions in the VTA and LH,
enhance human cerebral responses to visual food cues in the
modulates affective responses for food that coincide with NAc neu-
AMY, insula, orbitofrontal cortex (OFC) and striatum as measured
ral activity and regulates DA signalling and plasticity in the VTA to
by fMRI In this study, ghrelin treatment increased hunger
NAc pathway. On the one hand, leptin reduces the tonic firing of
ratings as a function of the degree of activation in the AMY and
DA neurons, while on the other, leptin is important for DA avail-
OFC to suggest that increased motivation for food induced by
ability, packaging and release. While these studies implicate the
ghrelin treatment is mediated by processes encoded in these re-
mesolimbic DA system and inputs from the LH as regions involved
gions. Collectively, the rodent and humans studies strongly impli-
in leptin action, additional investigations that measure reward
cate ghrelin in the modulation of the neural networks controlling
(e.g., operant responses for food), in particular, are required in or-
der to identify the neurons and mechanisms involved.
Human imaging studies have offered great insight into the
Insulin receptors are abundantly expressed throughout the
neural responses associated with motivation for food in different
brain, including the striatum and midbrain, and insulin is in-
metabolic states. Investigating the neural responses that are pre-
volved in the modulation of reward-relevant processes . Fig-
dictive of later food consumption, Batterham et al. measured neu-
lewicz et al. identified insulin receptors on DA neurons of the VTA
ral activity in response to the anorexigenic gut hormone PYY .
and SN These investigators report that insulin and leptin
These investigators found that when circulating PYY levels were
administration to the VTA increases immunoreactivity for phos-
low, as during the fasted state, changes in neural activity in the
phatidylinositol-3 (PI3) kinase, a signalling molecule activated
hypothalamus were predictive of later food intake. In contrast,
by insulin and leptin receptor signalling . In addition, they
changes in activity of the caudolateral OFC predicted feeding
show that VTA infusion of insulin and leptin attenuates the feed-
when PYY levels were high . These results demonstrate how
ing response induced by opioids in this region. Earlier studies
a postprandial satiety factor can switch neural controls of food in-
demonstrate the ability of insulin to decrease DA release in stria-
take from hypothalamic to corticolimbic regions and thereby
implicate corticolimbic processes in excessive caloric intake. Con-
hypoinsulinemia increases striatal DA release . Central insulin
sistent with this idea, Volkow, Wang and colleagues provide evi-
administration augments DAT mRNA levels in the SN and VTA
dence of reduced striatal D2 dopamine receptor binding in obese
whereas DAT mRNA levels are diminished during reduced
humans to suggest that there is reduced DA tone in these individ-
insulin signalling Carvelli et al. demonstrate the functional
uals Correspondingly, DS activation is attenuated in
actions of insulin to increase dopamine reuptake and facilitate the
obese humans, an effect that is even more pronounced in individ-
cell surface expression of DAT in vitro, and they provide evidence
uals with the A1 allele of Taq1A polymorphism which is associ-
that these effects are mediated by PI3-kinase signalling
ated with the D2 receptor gene and reduced striatal DA
Moreover, this group found that amphetamine-stimulated DA re-
signalling Interestingly, the results of several studies in hu-
lease in the striatum is impaired in insulin-deficient rats whereas
mans and rodents link diminished
insulin microinfusion into the striatum recovers this PI3-kinase
striatal DA signalling to hyperphagia and obesity. Thus, while
mediated effect . Together, the data suggest that insulin sup-
the consumption of palatable foods may stimulate striatal DA
presses striatal DA tone by increasing DA clearance. It remains to
be determined whether insulin directly influences DA neurotrans-
impairments in the amount of DA available for release may be
mission, although this possibility is supported by the observation
a factor promoting increased caloric intake.
that insulin interacts with opioids in the VTA to decrease food in-
As summarized in , information regarding the status of en-
take. Interestingly, opioids down-regulate the insulin-receptor
ergy reserves is being relayed to corticolimbic sites by the actions
substrate-2 (IRS2) thymoma viral proto-oncogene (Akt) pathway
of leptin, insulin and ghrelin to modulate DA and/or striatal cell
in DA neurons of the VTA to regulate the cellular and behavioural
signalling. It is possible that these and other hormones affect cor-
effects of morphine thus activation of IRS2-Akt pathway by
ticolimbic controls of food motivation by also signalling in up-
insulin may be responsible for attenuating opioid-induced
stream neural pathways in the hypothalamus and hindbrain.
Indeed, recent data shows that leptin targets LH-VTA projection
Like leptin and insulin, the gut-derived hormone ghrelin mod-
neurons to increase DA content . Moreover, insulin adminis-
ulates reward-relevant behaviour and targets DA neurons of the
tration in the ARC suppresses sucrose-reinforced instrumental
VTA. Ghrelin binds to growth hormone secretagogue receptors
responding . There remains several questions concerning the
(GHSR) to stimulate food intake and adiposity . GHSR recep-
precise mechanisms by which metabolic and central signals mod-
tors are expressed in several brain regions including the VTA
ulate DA activity states and release, which corticolimbic targets
Peripheral ghrelin enhances the locomotor-activating
and signalling molecules are affected and how these processes re-
effects of cocaine and conditioned place preference
lates to immediate and long-term behavioural changes. It also re-
Naleid and coworkers discovered that ghrelin injected in either
mains to be determined whether or not leptin, insulin and
S. Fulton / Frontiers in Neuroendocrinology 31 (2010) 85–103
ghrelin target the same DA neuronal subpopulations and, if so, if
nings for energy-rich food (e.g., ). Despite the complexity of
there are functional interactions between their respective receptor
these circuits, the use of cell-specific gene targeting, tract tracing,
signalling pathways. Finally, it is not yet clear to what extent the
pharmacological and electrophysiological approaches along with
signalling of these hormones in midbrain and limbic sites specifi-
precise behavioural measures offers great promise that future
cally influence the appetitive and/or consummatory aspects of
work will continue to tease apart the pathways and mechanisms
feeding. It could well be that hormonal sensing at these loci has
responsible for the rewarding effects of food.
more general actions to orient attention and motivation towardbehavioural actions that are compatible with the current energy
state of the animal.
The author thanks Thierry Alquier, Vincent Poitout and Marc
Prentki for valuable input on the manuscript.
The reward efficacy of food is not only influenced by fluctua-
tions in metabolic status but also by the palatability and post-ingestive consequences of foods. Thus, food reward can be en-
[1] E.L. Abel, Cannabis: effects on hunger and thirst, Behav. Biol. 15 (1975) 255–
hanced by the sensory qualities of food independent of energy de-
[2] J.E. Aberman, S.J. Ward, J.D. Salamone, Effects of dopamine antagonists and
mands and may provide a major basis for overeating and weight
accumbens dopamine depletions on time-constrained progressive-ratio
gain. One can deduce from such observations that neural processes
performance, Pharmacol. Biochem. Behav. 61 (1998) 341–348.
regulating motivation for food can override signals of satiety and
[3] A. Abizaid, Z.W. Liu, Z.B. Andrews, M. Shanabrough, E. Borok, J.D. Elsworth,
R.H. Roth, M.W. Sleeman, M.R. Picciotto, M.H. Tschop, X.B. Gao, T.L. Horvath,
adequate energy fuel and/or that impaired responses to such sig-
Ghrelin modulates the activity and synaptic input organization of midbrain
nals may develop that promote excessive food intake. There is evi-
dopamine neurons while promoting appetite, J. Clin. Invest. 116 (2006) 3229–
dence for both propositions. Palatable high-fat and -sugar foods
[4] G. Abrahamsen, Y. Berman, K. Carr, Curve-shift analysis of self-stimulation in
can generate neural responses in corticolimbic circuitry that
food-restricted rats: relationship between daily meal, plasma corticosterone
strengthen future behaviour directed towards these foods. Such
and reward sensitization, Brain Res. 695 (1995) 186–194.
mechanisms loom large in environmental settings inundated by
[5] G.C. Abrahamsen, K.D. Carr, Effects of corticosteroid synthesis inhibitors on
cues that summon up memories of eating and remind us how,
the sensitization of reward by food restriction, Brain Res. (1996) 39–48.
[6] A. Agmo, A. Galvan, B. Talamantes, Reward and reinforcement produced by
where and when we can get access to food. On the other hand, a
high-fat diet and resulting elevations in circulating adiposity sig-
neurotransmitters, Pharmacol. Biochem. Behav. 52 (1995) 403–414.
nals and nutrients can impair signalling mechanisms in the medi-
[7] S.T. Ahlers, M.K. Salander, Effects of repeated administration of corticotropin-
releasing factor on schedule-controlled behavior in rats, Pharmacol. Biochem.
obasal hypothalamus and thereby weaken catabolic responses
Behav. 44 (1993) 375–380.
. Such impaired responses to anorexigenic signals may
[8] S.T. Ahlers, M.K. Salander, D. Shurtleff, J.R. Thomas, Tyrosine pretreatment
represent another means whereby motivation for food may be in-
alleviates suppression of schedule-controlled responding produced bycorticotropin releasing factor (CRF) in rats, Brain Res. Bull. 29 (1992)
creased by high-fat and -sugar foods.
Future research will benefit from considering the important
[9] S. Ahn, A.G. Phillips, Dopaminergic correlates of sensory-specific satiety in the
influence of environmental variables and their interaction with
medial prefrontal cortex and nucleus accumbens of the rat, J. Neurosci. 19(1999) RC29.
biological and genetic components on the type and amount of food
[10] K.D. Alex, E.A. Pehek, Pharmacologic mechanisms of serotonergic regulation
we choose to eat. For example, epidemiological evidence suggests
of dopamine neurotransmission, Pharmacol. Ther. 113 (2007) 296–320.
that poor quality food options in conjunction with limited eco-
[11] G.E. Alexander, M.D. Crutcher, Functional architecture of basal ganglia
circuits: neural substrates of parallel processing, Trends Neurosci. 13
nomic resources contribute to increased risk for obesity in socio-
(1990) 266–271.
[12] G.E. Alexander, M.R. DeLong, P.L. Strick, Parallel organization of functionally
settings have been modeled in rodent experiments by manipulat-
segregated circuits linking basal ganglia and cortex, Annu. Rev. Neurosci. 9
ing the availability of food alternatives. In rats it has been shown
(1986) 357–381.
[13] B. Anand, J. Brobeck, Hypothalamic control of food intake in rats and cats,
that increasing the number of containers of high-fat and -carbohy-
Proc. Soc. Exp. Biol. Med. 77 (1951) 323–324.
drate food in the cage elevates caloric intake relative to conditions
[14] M. Apfelbaum, A. Mandenoff, Naltrexone suppresses hyperphagia induced in
where fewer containers, but equal amounts, of these foods are
the rat by a highly palatable diet, Pharmacol. Biochem. Behav. 15 (1981) 89–91.
available . Similarly, economic conditions are modeled in ro-
[15] P. Apicella, Tonically active neurons in the primate striatum and their role in
dent studies by influencing the price (response requirement) of
the processing of information about motivationally relevant events, Eur. J.
food and/or limiting the context in which food alternatives are
Neurosci. 16 (2002) 2017–2026.
[16] A. Arvanitogiannis, C. Flores, J.G. Pfaus, P. Shizgal, Increased ipsilateral
available For instance, appetite for fat solution in rats
expression of Fos following lateral hypothalamic self-stimulation, Brain Res.
is greatly affected by the price of the fat reinforcer, especially when
720 (1996) 148–154.
other palatable food alternatives are available Thus, in envi-
[17] A. Arvanitogiannis, P. Shizgal, The reinforcement mountain: allocation of
behavior as a function of the rate and intensity of rewarding brain
ronments in which the cost of high-fat and sugar foods is relatively
stimulation, Behav. Neurosci. 122 (2008) 1126–1138.
low and financial resources are limited, it is increasingly evident
[18] A. Arvanitogiannis, T.M. Tzschentke, L. Riscaldino, R.A. Wise, P. Shizgal, Fos
that we need to unravel the neural mechanisms that process the
expression following self-stimulation of the medial prefrontal cortex, Behav.
Brain Res. 107 (2000) 123–132.
rewarding effects of palatable foods in the context of modern social
[19] N.M. Avena, M.E. Bocarsly, P. Rada, A. Kim, B.G. Hoebel, After daily bingeing
and economic climates.
on a sucrose solution, food deprivation induces anxiety and accumbens
Neurons in the VTA, LH and ARC are well-positioned to relay
dopamine/acetylcholine imbalance, Physiol. Behav. 94 (2008) 309–315.
metabolic information to corticolimbic controls, but clearly more
[20] A.V. Azzara, R.J. Bodnar, A.R. Delamater, A. Sclafani, D1 but not D2 dopamine
receptor antagonism blocks the acquisition of a flavor preference conditioned
remains to be uncovered about the pathways and signalling mole-
by intragastric carbohydrate infusions, Pharmacol. Biochem. Behav. 68 (2001)
cules by which this information is conveyed. Metabolic informa-
tion is channelled to the striatum, especially the NAc shell,
[21] V.P. Bakshi, A.E. Kelley, Feeding induced by opioid stimulation of the ventral
striatum: role of opiate receptor subtypes, J. Pharmacol. Exp. Ther. 265 (1993)
where DA, Ach, opioids and endocannabinoids signals interact to
modulate affective and goal-directed responses for food. Finally,
[22] B.A. Baldo, L. Gual-Bonilla, K. Sijapati, R.A. Daniel, C.F. Landry, A.E. Kelley,
great headway has been made in determining the molecular mech-
Activation of a subpopulation of orexin/hypocretin-containing hypothalamicneurons by GABAA receptor-mediated inhibition of the nucleus accumbens
anisms tied to long-lasting behavioural responses for drug rewards
shell, but not by exposure to a novel environment, Eur. J. Neurosci. 19 (2004)
and recent work is shedding light on similar molecular underpin-
S. Fulton / Frontiers in Neuroendocrinology 31 (2010) 85–103
[23] B.A. Baldo, A.E. Kelley, Discrete neurochemical coding of distinguishable
[53] M. Cabanac, Palatability of food and the ponderostat, Ann. N.Y. Acad. Sci. 575
motivational processes: insights from nucleus accumbens control of feeding,
(1989) 340–351 (discussion 352).
Psychopharmacology (Berl.) 191 (2007) 439–459.
[54] M. Cabanac, A. Dagnault, D. Richard, The food-hoarding threshold is not
[24] A.E. Baldwin, K. Sadeghian, A.E. Kelley, Appetitive instrumental learning
raised by acute intraventricular NPY in male rats, Physiol. Behav. 61 (1997)
requires coincident activation of NMDA and dopamine D1 receptors within
the medial prefrontal cortex, J. Neurosci. 22 (2002) 1063–1071.
[55] M. Cabanac, R. Duclaux, N.H. Spector, Sensory feedback in regulation of body
[25] B. Balleine, A. Dickinson, Role of cholecystokinin in the motivational control
weight: is there a ponderostat?, Nature 229 (1971) 125–127
of instrumental action in rats, Behav. Neurosci. 108 (1994) 590–605.
[56] M. Cabanac, A.H. Swiergiel, Rats eating and hoarding as a function of body
[26] M.F. Barbano, M. Le Saux, M. Cador, Involvement of dopamine, opioids in the
weight and cost of foraging, Am. J. Physiol. 257 (1989) R952–R957.
motivation to eat: influence of palatability, homeostatic state, and behavioral
[57] S. Cabeza de Vaca, S. Holiman, K. Carr, A search for the metabolic signals that
paradigms, Psychopharmacology (Berl.) 203 (2009) 475–487.
sensitize lateral hypothalamic self-stimulation in food restricted rats, Physiol.
[27] A.A. Bari, R.C. Pierce, D1-like and D2 dopamine receptor antagonists
Behav. 64 (1998) 251–260.
administered into the shell subregion of the rat nucleus accumbens
[58] W.A. Carlezon Jr., R.A. Wise, Rewarding actions of phencyclidine and related
decrease cocaine, but not food, reinforcement, Neuroscience 135 (2005)
drugs in nucleus accumbens shell and frontal cortex, J. Neurosci. 16 (1996)
[28] V. Bassareo, G. Di Chiara, Differential influence of associative and
[59] K. Carr, V. Papadouka, The role of mulyiple opioid receptors in the
nonassociative learning mechanisms on the responsiveness of prefrontal
potentiation of reward by food restriction, Brain Res. 639 (1994) 253–260.
and accumbal dopamine transmission to food stimuli in rats fed ad libitum, J.
[60] K.D. Carr, Streptozotocin-induced diabetes produces a naltrexone-reversible
Neurosci. 17 (1997) 851–861.
lowering of self-stimulation threshold, Brain Res. 664 (1994) 211–214.
[29] V. Bassareo, G. Di Chiara, Modulation of feeding-induced activation of
[61] K.D. Carr, G. Kim, S. Cabeza de Vaca, Hypoinsulinemia may mediate the
mesolimbic dopamine transmission by appetitive stimuli and its relation to
motivational state, Eur. J. Neurosci. 11 (1999) 4389–4397.
streptozotocin-induced diabetes, Brain Res. 863 (2000) 160–168.
[30] R.L. Batterham, M.A. Cohen, S.M. Ellis, C.W. Le Roux, D.J. Withers, G.S. Frost,
[62] K.D. Carr, T.D. Wolinsky, Chronic food restriction and weight loss produce
M.A. Ghatei, S.R. Bloom, Inhibition of food intake in obese subjects by peptide
opioid facilitation of perifornical hypothalamic self-stimulation, Brain Res.
YY3-36, New Engl. J. Med. 349 (2003) 941–948.
607 (1993) 141–148.
[31] R.L. Batterham, M.A. Cowley, C.J. Small, H. Herzog, M.A. Cohen, C.L. Dakin,
[63] L. Carvelli, J.A. Moron, K.M. Kahlig, J.V. Ferrer, N. Sen, J.D. Lechleiter, L.M. Leeb-
A.M. Wren, A.E. Brynes, M.J. Low, M.A. Ghatei, R.D. Cone, S.R. Bloom, Gut
Lundberg, G. Merrill, E.M. Lafer, L.M. Ballou, T.S. Shippenberg, J.A. Javitch, R.Z.
hormone PYY(3–36) physiologically inhibits food intake, Nature 418 (2002)
Lin, A. Galli, PI 3-kinase regulation of dopamine uptake, J. Neurochem. 81
(2002) 859–869.
[32] R.L. Batterham, D.H. ffytche, J.M. Rosenthal, F.O. Zelaya, G.J. Barker, D.J.
[64] R. Caulliez, M.J. Meile, S. Nicolaidis, A lateral hypothalamic D1 dopaminergic
Withers, S.C. Williams, PYY modulation of cortical and hypothalamic brain
mechanism in conditioned taste aversion, Brain Res. 729 (1996) 234–245.
areas predicts feeding behaviour in humans, Nature 450 (2007) 106–109.
[65] M.A. Cenci, P. Kalen, R.J. Mandel, A. Bjorklund, Regional differences in the
[33] D. Belin, S. Jonkman, A. Dickinson, T.W. Robbins, B.J. Everitt, Parallel and
regulation of dopamine and noradrenaline release in medial frontal cortex,
interactive learning processes within the basal ganglia: relevance for the
nucleus accumbens and caudate-putamen: a microdialysis study in the rat,
understanding of addiction, Behav. Brain Res. 199 (2009) 89–102.
Brain Res. 581 (1992) 217–228.
[34] R.J. Beninger, F. Bellisle, P.M. Milner, Schedule control of behavior reinforced
[66] J.F. Cheer, K.M. Wassum, M.L. Heien, P.E. Phillips, R.M. Wightman,
by electrical stimulation of the brain, Science 196 (1977) 547–549.
Cannabinoids enhance subsecond dopamine release in the nucleus
[35] L.L. Bernardis, L.L. Bellinger, The lateral hypothalamic area revisited: ingestive
accumbens of awake rats, J. Neurosci. 24 (2004) 4393–4400.
behavior, Neurosci. Biobehav. Rev. 20 (1996) 189–287.
[67] S. Cheeta, S. Brooks, P. Willner, Effects of reinforcer sweetness and the D2/D3
[36] K.C. Berridge, Food reward: brain substrates of wanting and liking, Neurosci.
antagonist raclopride on progressive ratio operant performance, Behav.
Biobehav. Rev. 20 (1996) 1–25.
Pharmacol. 6 (1995) 127–132.
[68] J. Cleary, D.T. Weldon, E. O'Hare, C. Billington, A.S. Levine, Naloxone effects on
microstructure of affective taste reactivity patterns, Neurosci. Biobehav.
sucrose-motivated behavior, Psychopharmacology (Berl.) 126 (1996) 110–
Rev. 24 (2000) 173–198.
[38] K.C. Berridge, T.E. Robinson, What is the role of dopamine in reward: hedonic
[69] D.J. Clegg, E.L. Air, S.C. Woods, R.J. Seeley, Eating elicited by orexin-a, but not
impact, reward learning, or incentive salience?, Brain Res Brain Res. Rev. 28
melanin-concentrating hormone, is opioid mediated, Endocrinology 143
(1998) 309–369.
(2002) 2995–3000.
[39] K.C. Berridge, I.L. Venier, T.E. Robinson, Taste reactivity analysis of 6-
[70] K.L. Conover, P. Shizgal, Competition and summation between rewarding
hydroxydopamine-induced aphagia: implications for arousal and anhedonia
effects of sucrose and lateral hypothalamic stimulation in the rat, Behav.
hypotheses of dopamine function, Behav. Neurosci. 103 (1989) 36–45.
Neurosci. 108 (1994) 537–548.
[40] C. Bielajew, P. Shizgal, Evidence implicating descending fibers in self-
[71] K.L. Conover, P. Shizgal, Differential effects of postingestive feedback on the
stimulation of the medial forebrain bundle, J. Neurosci. 6 (1986) 919–929.
reward value of sucrose and lateral hypothalamic stimulation in the rat,
[41] M. Bishop, S. Elder, R. Heath, Intra-cranial self-stimulation in man, Science
Behav. Neurosci. 108 (1994) 559–572.
140 (1963) 394–396.
[72] K.L. Conover, B. Woodside, P. Shizgal, Effects of sodium depletion on
[42] J.E. Blundell, L.J. Herberg, Relative effects of nutritional deficit and deprivation
competition and summation between rewarding effects of salt and lateral
period on rate of electrical self-stimulation of lateral hypothalamus, Nature
hypothalamic stimulation in the rat, Behav. Neurosci. 108 (1994) 549–
219 (1968) 627–628.
[43] S.L. Borgland, E. Storm, A. Bonci, Orexin B/hypocretin 2 increases
[73] D. Corbett, R.A. Wise, Intracranial self-stimulation in relation to the ascending
glutamatergic transmission to ventral tegmental area neurons, Eur. J.
noradrenergic fiber systems of the pontine tegmentum and caudal midbrain:
Neurosci. 28 (2008) 1545–1556.
a moveable electrode mapping study, Brain Res. 177 (1979) 423–436.
[44] S.L. Borgland, S.A. Taha, F. Sarti, H.L. Fields, A. Bonci, Orexin A in the VTA is
[74] T.L. Davidson, K. Chan, L.E. Jarrard, S.E. Kanoski, D.J. Clegg, S.C. Benoit,
critical for the induction of synaptic plasticity and behavioral sensitization to
Contributions of the hippocampus and medial prefrontal cortex to energy and
cocaine, Neuron 49 (2006) 589–601.
body weight regulation, Hippocampus 19 (2009) 235–252.
[45] M. Boules, I. Iversen, A. Oliveros, A. Shaw, K. Williams, J. Robinson, P.
[75] T.L. Davidson, S.E. Kanoski, L.A. Schier, D.J. Clegg, S.C. Benoit, A potential role
Fredrickson, E. Richelson, The neurotensin receptor agonist NT69L suppresses
for the hippocampus in energy intake and body weight regulation, Curr. Opin.
sucrose-reinforced operant behavior in the rat, Brain Res. 1127 (2007) 90–98.
Pharmacol. 7 (2007) 613–616.
[46] K. Brebner, W. Froestl, M. Andrews, R. Phelan, D.C. Roberts, The GABA(B)
[76] L.M. Davis, M. Michaelides, L.J. Cheskin, T.H. Moran, S. Aja, P.A. Watkins, Z. Pei,
C. Contoreggi, K. McCullough, B. Hope, G.J. Wang, N.D. Volkow, P.K. Thanos,
demonstration using a progressive ratio and a discrete trials procedure,
Bromocriptine administration reduces hyperphagia and adiposity and
Neuropharmacology 38 (1999) 1797–1804.
differentially affects dopamine D2 receptor and transporter binding in
[47] K. Brennan, D.C. Roberts, H. Anisman, Z. Merali, Individual differences in
leptin-receptor-deficient Zucker rats and rats with diet-induced obesity,
sucrose consumption in the rat: motivational and neurochemical correlates
Neuroendocrinology 89 (2009) 152–162.
of hedonia, Psychopharmacology (Berl.) 157 (2001) 269–276.
[77] I.E. de Araujo, A.J. Oliveira-Maia, T.D. Sotnikova, R.R. Gainetdinov, M.G. Caron,
[48] E. Briese, M. Quijada, Sugar solutions taste better (positive alliesthesia) after
M.A. Nicolelis, S.A. Simon, Food reward in the absence of taste receptor
insulin (proceedings), J. Physiol. 285 (1978) 20P–21P.
signaling, Neuron 57 (2008) 930–941.
[49] E. Briese, M. Quijada, Positive alliesthesia after insulin, Experientia 35 (1979)
[78] J. Deutsch, L. DiCiara, Hunger and extiction in intracranial self-stimulation, J.
Comp. Physiol. Psychol. 63 (1967) 344–347.
[50] D.R. Britton, G.F. Koob, J. Rivier, W. Vale, Intraventricular corticotropin-
[79] D.P. Devine, P. Leone, R.A. Wise, Mesolimbic dopamine neurotransmission is
releasing factor enhances behavioral effects of novelty, Life Sci. 31 (1982)
increased by administration of mu-opioid receptor antagonists, Eur. J.
Pharmacol. 243 (1993) 55–64.
[51] P.A. Broderick, J.H. Jacoby, Central monoamine dysfunction in diabetes:
[80] G. Di Chiara, Nucleus accumbens shell and core dopamine: differential role in
psychotherapeutic implications: electroanalysis by voltammetry, Acta
behavior and addiction, Behav. Brain Res. 137 (2002) 75–114.
Physiol. Pharmacol. Latinoam 39 (1989) 211–225.
[81] V. Di Marzo, I. Matias, Endocannabinoid control of food intake and energy
[52] C.M. Brown, P.J. Fletcher, D.V. Coscina, Neuropeptide Y-induced operant
balance, Nat. Neurosci. 8 (2005) 585–589.
responding for sucrose is not mediated by dopamine, Peptides 19 (1998)
[82] T.G. Doyle, K.C. Berridge, B.A. Gosnell, Morphine enhances hedonic taste
palatability in rats, Pharmacol. Biochem. Behav. 46 (1993) 745–749.
S. Fulton / Frontiers in Neuroendocrinology 31 (2010) 85–103
[83] C. Duarte, G. Biala, C. Le Bihan, M. Hamon, M.H. Thiebot, Respective roles of
[113] M. Gallagher, A.A. Chiba, The amygdala and emotion, Curr. Opin. Neurobiol. 6
dopamine D2 and D3 receptors in food-seeking behaviour in rats,
(1996) 221–227.
Psychopharmacology (Berl.) 166 (2003) 19–32.
[114] C.R. Gallistel, G. Beagley, Specificity of brain stimulation reward in the rat, J.
[84] C.F. Elias, C.B. Saper, E. Maratos-Flier, N.A. Tritos, C. Lee, J. Kelly, J.B. Tatro, G.E.
Comp. Physiol. Psychol. 76 (1971) 199–205.
Hoffman, M.M. Ollmann, G.S. Barsh, T. Sakurai, M. Yanagisawa, J.K. Elmquist,
[115] C.R. Gallistel, D. Karras, Pimozide and amphetamine have opposing effects on
Chemically defined projections linking the mediobasal hypothalamus and the
the reward summation function, Pharmacol. Biochem. Behav. 20 (1984) 73–
lateral hypothalamic area, J. Comp. Neurol. 402 (1998) 442–459.
[85] J.K. Elmquist, C. Bjorbaek, R.S. Ahima, J.S. Flier, C.B. Saper, Distributions of
[116] C.R. Gallistel, P. Shizgal, J.S. Yeomans, A portrait of the substrate for self-
leptin receptor mRNA isoforms in the rat brain, J. Comp. Neurol. 395 (1998)
stimulation, Psychol. Rev. 88 (1981) 228–273.
[117] P.A. Garris, E.L. Ciolkowski, P. Pastore, R.M. Wightman, Efflux of dopamine
[86] R. Emmers, Modifications of sensory modality codes by stimuli of graded
from the synaptic cleft in the nucleus accumbens of the rat brain, J. Neurosci.
intensity in the cat thalamus, Brain Res. 21 (1970) 91–104.
14 (1994) 6084–6093.
[87] Epicurus, The Extant Remains, The Clarendon Press, Oxford, 1926.
[118] J.T. Gass, M.P. Osborne, N.L. Watson, J.L. Brown, M.F. Olive, MGluR5
[88] R. Esposito, C. Kornetsky, Morphine lowering of self-stimulation thresholds:
antagonism attenuates methamphetamine reinforcement and prevents
lack of tolerance with long-term administration, Science 195 (1977) 189–
Neuropsychopharmacology 34 (2009) 820–833.
[89] R.U. Esposito, A.H. Motola, C. Kornetsky, Cocaine: acute effects on
[119] B.M. Geiger, G.G. Behr, L.E. Frank, A.D. Caldera-Siu, M.C. Beinfeld, E.G.
reinforcement thresholds for self-stimulation behavior to the medial
Kokkotou, E.N. Pothos, Evidence for defective mesolimbic dopamine
forebrain bundle, Pharmacol. Biochem. Behav. 8 (1978) 437–439.
exocytosis in obesity-prone rats, Faseb J. 22 (2008) 2740–2746.
[90] J. Fadel, A.Y. Deutch, Anatomical substrates of orexin–dopamine interactions:
lateral hypothalamic projections to the ventral tegmental area, Neuroscience
psychostimulants, and stress – emphasis on neuroanatomical substrates,
111 (2002) 379–387.
Peptides 27 (2006) 2364–2384.
[91] M. Fantino, M. Cabanac, Body weight regulation with a proportional hoarding
[121] D. Georgescu, R.M. Sears, J.D. Hommel, M. Barrot, C.A. Bolanos, D.J. Marsh,
response in the rat, Physiol. Behav. 24 (1980) 939–942.
M.A. Bednarek, J.A. Bibb, E. Maratos-Flier, E.J. Nestler, R.J. DiLeone, The
[92] M. Fantino, J. Hosotte, M. Apfelbaum, An opioid antagonist, naltrexone,
hypothalamic neuropeptide melanin-concentrating hormone acts in the
reduces preference for sucrose in humans, Am. J. Physiol. 251 (1986) R91–
nucleus accumbens to modulate feeding behavior and forced-swim
performance, J. Neurosci. 25 (2005) 2933–2940.
[93] I.S. Farooqi, E. Bullmore, J. Keogh, J. Gillard, S. O'Rahilly, P.C. Fletcher, Leptin
regulates striatal regions and human eating behavior, Science 317 (2007)
compartmental distribution of calcium-binding protein and parvalbumin in
the basal ganglia of the rat and monkey, Proc. Natl. Acad. Sci. USA 82 (1985)
[94] A. Faure, U. Haberland, F. Conde, N. El Massioui, Lesion to the nigrostriatal
dopamine system disrupts stimulus-response habit formation, J. Neurosci. 25
[123] C.R. Gerfen, T.M. Engber, L.C. Mahan, Z. Susel, T.N. Chase, F.J. Monsma Jr., D.R.
(2005) 2771–2780.
Sibley, D1 and D2 dopamine receptor-regulated gene expression of
[95] S. Fenu, V. Bassareo, G. Di Chiara, A role for dopamine D1 receptors of the
striatonigral and striatopallidal neurons, Science 250 (1990) 1429–1432.
nucleus accumbens shell in conditioned taste aversion learning, J. Neurosci.
[124] U.E. Ghitza, S.M. Gray, D.H. Epstein, K.C. Rice, Y. Shaham, The anxiogenic drug
21 (2001) 6897–6904.
yohimbine reinstates palatable food seeking in a rat relapse model: a role of
[96] D.P. Figlewicz, J. Bennett, S.B. Evans, K. Kaiyala, A.J. Sipols, S.C. Benoit,
CRF1 receptors, Neuropsychopharmacology 31 (2006) 2188–2196.
Intraventricular insulin and leptin reverse place preference conditioned with
[125] U.E. Ghitza, S.G. Nair, S.A. Golden, S.M. Gray, J.L. Uejima, J.M. Bossert, Y.
high-fat diet in rats, Behav. Neurosci. 118 (2004) 479–487.
Shaham, Peptide YY3–36 decreases reinstatement of high-fat food seeking
[97] D.P. Figlewicz, J.L. Bennett, S. Aliakbari, A. Zavosh, A.J. Sipols, Insulin acts at
during dieting in a rat relapse model, J. Neurosci. 27 (2007) 11522–
different CNS sites to decrease acute sucrose intake and sucrose self-
administration in rats, Am. J. Physiol. Regul. Integr. Comp. Physiol. 295
[126] M.J. Glass, M. Grace, J.P. Cleary, C.J. Billington, A.S. Levine, Potency of
(2008) R388–R394.
naloxone's anorectic effect in rats is dependent on diet preference, Am. J.
[98] D.P. Figlewicz, J.L. Bennett, A.M. Naleid, C. Davis, J.W. Grimm, Intraventricular
Physiol. 271 (1996) R217–R221.
insulin and leptin decrease sucrose self-administration in rats, Physiol. Behav.
[127] M.J. Glass, E. O'Hare, J.P. Cleary, C.J. Billington, A.S. Levine, The effect of
89 (2006) 611–616.
[99] D.P. Figlewicz, S.B. Evans, J. Murphy, M. Hoen, D.G. Baskin, Expression of
Psychopharmacology (Berl.) 141 (1999) 378–384.
receptors for insulin and leptin in the ventral tegmental area/substantia nigra
[128] N.E. Goeders, G.F. Guerin, Effects of the CRH receptor antagonist CP-154, 526
(VTA/SN) of the rat, Brain Res. 964 (2003) 107–115.
[100] D.P. Figlewicz, A. MacDonald Naleid, A.J. Sipols, Modulation of food reward by
Neuropsychopharmacology 23 (2000) 577–586.
adiposity signals, Physiol. Behav. 91 (2007) 473–478.
[129] N.E. Goeders, J.E. Smith, Cortical dopaminergic involvement in cocaine
[101] D.P. Figlewicz, T.A. Patterson, L.B. Johnson, A. Zavosh, P.A. Israel, P. Szot,
reinforcement, Science 221 (1983) 773–775.
Dopamine transporter mRNA is increased in the CNS of Zucker fatty (fa/fa)
[130] N.E. Goeders, J.E. Smith, Parameters of intracranial self-administration of
rats, Brain Res. Bull. 46 (1998) 199–202.
cocaine into the medial prefrontal cortex, NIDA Res. Monogr. 55 (1984) 132–
[102] D.P. Figlewicz, P. Szot, M. Chavez, S.C. Woods, R.C. Veith, Intraventricular
insulin increases dopamine transporter mRNA in rat VTA/substantia nigra,
[131] P.S. Goldman-Rakic, Development of cortical circuitry and cognitive function,
Brain Res. 644 (1994) 331–334.
Child Dev. 58 (1987) 601–622.
[103] P.J. Fletcher, A. Azampanah, K.M. Korth, Activation of 5-HT(1B) receptors in
[132] P.S. Goldman-Rakic, L.D. Selemon, New frontiers in basal ganglia research.
the nucleus accumbens reduces self-administration of amphetamine on a
Introduction, Trends Neurosci. 13 (1990) 241–244.
progressive ratio schedule, Pharmacol. Biochem. Behav. 71 (2002) 717–
[133] F.G. Gonon, M.J. Buda, Regulation of dopamine release by impulse flow and by
autoreceptors as studied by in vivo voltammetry in the rat striatum,
[104] P.B. Ford, D.A. Dzewaltowski, Disparities in obesity prevalence due to
Neuroscience 14 (1985) 765–774.
variation in the retail food environment: three testable hypotheses, Nutr.
[134] B.A. Gosnell, J.E. Morley, A.S. Levine, A comparison of the effects of
Rev. 66 (2008) 216–228.
corticotropin releasing factor and sauvagine on food intake, Pharmacol.
[105] G. Fouriezos, R.A. Wise, Pimozide-induced extinction of intracranial self-
Biochem. Behav. 19 (1983) 771–775.
stimulation: response patterns rule out motor or performance deficits, Brain
[135] A.A. Grace, The tonic/phasic model of dopamine system regulation: its
Res. 103 (1976) 377–380.
relevance for understanding how stimulant abuse can alter basal ganglia
[106] D.E. Freed, L. Green, A behavioral economic analysis of fat appetite in rats,
function, Drug Alcohol Depend. 37 (1995) 111–129.
Appetite 31 (1998) 333–349.
[136] A.A. Grace, B.S. Bunney, The control of firing pattern in nigral dopamine
[107] S. Fulton, P. Pissios, R.P. Manchon, L. Stiles, L. Frank, E.N. Pothos, E. Maratos-
neurons: burst firing, J. Neurosci. 4 (1984) 2877–2890.
Flier, J.S. Flier, Leptin regulation of the mesoaccumbens dopamine pathway,
[137] A.A. Grace, B.S. Bunney, The control of firing pattern in nigral dopamine
Neuron 51 (2006) 811–822.
neurons: single spike firing, J. Neurosci. 4 (1984) 2866–2876.
[108] S. Fulton, D. Richard, B. Woodside, P. Shizgal, Interaction of CRH and energy
[138] A.A. Grace, S.B. Floresco, Y. Goto, D.J. Lodge, Regulation of firing of
balance in the modulation of brain stimulation reward, Behav. Neurosci. 116
dopaminergic neurons and control of goal-directed behaviors, Trends
(2002) 651–659.
Neurosci. 30 (2007) 220–227.
[109] S. Fulton, D. Richard, B. Woodside, P. Shizgal, Food restriction and leptin
[139] A. Gratton, B.J. Hoffer, G.A. Gerhardt, Effects of electrical stimulation of brain
impact brain reward circuitry in lean and obese Zucker rats, Behav. Brain Res.
reward sites on release of dopamine in rat: an in vivo electrochemical study,
155 (2004) 319–329.
Brain Res. Bull. 21 (1988) 319–324.
[110] S. Fulton, B. Woodside, P. Shizgal, Modulation of brain reward circuitry by
[140] G.A. Graveland, M. DiFiglia, The frequency and distribution of medium-sized
leptin, Science 287 (2000) 125–128.
neurons with indented nuclei in the primate and rodent neostriatum, Brain
[111] S. Fulton, B. Woodside, P. Shizgal, Does neuropeptide Y contribute to the
Res. 327 (1985) 307–311.
modulation of brain stimulation reward by chronic food restriction?, Behav
[141] A.M. Graybiel, R.W. Baughman, F. Eckenstein, Cholinergic neuropil of the
Brain Res. 134 (2002) 157–164.
striatum observes striosomal boundaries, Nature 323 (1986) 625–627.
[112] S. Fulton, B. Woodside, P. Shizgal, Potentiation of brain stimulation reward by
[142] A.J. Grottick, P.J. Fletcher, G.A. Higgins, Studies to investigate the role of 5-
weight loss: evidence for functional heterogeneity in brain reward circuitry,
HT(2C) receptors on cocaine- and food-maintained behavior, J. Pharmacol.
Behav. Brain Res. 174 (2006) 56–63.
Exp. Ther. 295 (2000) 1183–1191.
S. Fulton / Frontiers in Neuroendocrinology 31 (2010) 85–103
[143] N.E. Grunberg, Nicotine as a psychoactive drug: appetite regulation,
[174] D. Huston-Lyons, C. Kornetsky, Effects of nicotine on the threshold for
Psychopharmacol. Bull. 22 (1986) 875–881.
rewarding brain stimulation in rats, Pharmacol. Biochem. Behav. 41 (1992)
[144] X.M. Guan, H. Yu, O.C. Palyha, K.K. McKee, S.D. Feighner, D.J. Sirinathsinghji,
R.G. Smith, L.H. Van der Ploeg, A.D. Howard, Distribution of mRNA encoding
[175] S.E. Hyman, R.C. Malenka, E.J. Nestler, Neural mechanisms of addiction: the
the growth hormone secretagogue receptor in brain and peripheral tissues,
role of reward-related learning and memory, Annu. Rev. Neurosci. 29 (2006)
Brain Res. Mol. Brain Res. 48 (1997) 23–29.
[145] S.N. Haber, J.L. Fudge, N.R. McFarland, Striatonigrostriatal pathways in
[176] M.A. Hynes, M. Gallagher, K.V. Yacos, Systemic and intraventricular naloxone
primates form an ascending spiral from the shell to the dorsolateral
administration: effects on food and water intake, Behav. Neural Biol. 32
striatum, J. Neurosci. 20 (2000) 2369–2382.
(1981) 334–342.
[146] A. Hajnal, B.C. De Jonghe, M. Covasa, Dopamine D2 receptors contribute to
[177] S. Ikemoto, Dopamine reward circuitry: two projection systems from the
increased avidity for sucrose in obese rats lacking CCK-1 receptors,
ventral midbrain to the nucleus accumbens–olfactory tubercle complex,
Neuroscience 148 (2007) 584–592.
Brain Res. Rev. 56 (2007) 27–78.
[147] A. Hajnal, M. Szekely, R. Galosi, L. Lenard, Accumbens cholinergic
[178] M. Imaizumi, M. Takeda, T. Fushiki, Effects of oil intake in the conditioned
interneurons play a role in the regulation of body weight and metabolism,
place preference test in mice, Brain Res. 870 (2000) 150–156.
Physiol. Behav. 70 (2000) 95–103.
[179] J.N. Jaworski, S.T. Hansen, M.J. Kuhar, G.P. Mark, Injection of CART (cocaine-
[148] M.L. Hakansson, H. Brown, N. Ghilardi, R.C. Skoda, B. Meister, Leptin receptor
and amphetamine-regulated transcript) peptide into the nucleus accumbens
immunoreactivity in chemically defined target neurons of the hypothalamus,
reduces cocaine self-administration in rats, Behav. Brain Res. 191 (2008)
J. Neurosci. 18 (1998) 559–572.
[149] S. Hamill, J.T. Trevitt, K.L. Nowend, B.B. Carlson, J.D. Salamone, Nucleus
[180] E. Jerlhag, Systemic administration of ghrelin induces conditioned place
accumbens dopamine depletions and time-constrained progressive ratio
preference and stimulates accumbal dopamine, Addict. Biol. 13 (2008) 358–
performance: effects of different ratio requirements, Pharmacol. Biochem.
Behav. 64 (1999) 21–27.
[181] E. Jerlhag, E. Egecioglu, S.L. Dickson, M. Andersson, L. Svensson, J.A. Engel,
[150] J. Hao, S. Cabeza de Vaca, Y. Pan, K.D. Carr, Effects of central leptin infusion on
Ghrelin stimulates locomotor activity and accumbal dopamine-overflow via
the reward-potentiating effect of D-amphetamine, Brain Res. 1087 (2006)
central cholinergic systems in mice. implications for its involvement in brain
reward, Addict. Biol. 11 (2006) 45–54.
[151] V. Haroutunian, P. Knott, K.L. Davis, Effects of mesocortical dopaminergic
[182] E. Jerlhag, E. Egecioglu, S.L. Dickson, A. Douhan, L. Svensson, J.A. Engel,
lesions upon subcortical dopaminergic function, Psychopharmacol. Bull. 24
Ghrelin administration into tegmental areas stimulates locomotor activity
(1988) 341–344.
and increases extracellular concentration of dopamine in the nucleus
[152] J. Havrankova, J. Roth, M. Brownstein, Insulin receptors are widely distributed
accumbens, Addict. Biol. 12 (2007) 6–16.
in the central nervous system of the rat, Nature 272 (1978) 827–829.
[183] D.C. Jewett, J. Cleary, A.S. Levine, D.W. Schaal, T. Thompson, Effects of
[153] M.D. Hayward, M.J. Low, The effect of naloxone on operant behavior for food
neuropeptide Y, insulin, 2-deoxyglucose, and food deprivation on food-
reinforcers in DBA/2 mice, Brain Res. Bull. 56 (2001) 537–543.
motivated behavior, Psychopharmacology (Berl.) 120 (1995) 267–271.
[154] M.D. Hayward, M.J. Low, The contribution of endogenous opioids to food
[184] Y.H. Jo, D. Wiedl, L.W. Role, Cholinergic modulation of appetite-related
reward is dependent on sex and background strain, Neuroscience 144 (2007)
synapses in mouse lateral hypothalamic slice, J. Neurosci. 25 (2005) 11133–
[155] M.D. Hayward, J.E. Pintar, M.J. Low, Selective reward deficit in mice lacking
[185] M. Joshua, A. Adler, R. Mitelman, E. Vaadia, H. Bergman, Midbrain
beta-endorphin and enkephalin, J. Neurosci. 22 (2002) 8251–8258.
dopaminergic neurons and striatal cholinergic interneurons encode the
[156] M.D. Hayward, A. Schaich-Borg, J.E. Pintar, M.J. Low, Differential involvement
difference between reward and aversive events at different epochs of
of endogenous opioids in sucrose consumption and food reinforcement,
probabilistic classical conditioning trials, J. Neurosci. 28 (2008) 11673–
Pharmacol. Biochem. Behav. 85 (2006) 601–611.
[157] K.A. Helm, P. Rada, B.G. Hoebel, Cholecystokinin combined with serotonin in
[186] S.A. Josselyn, R.J. Beninger, Neuropeptide Y: intraaccumbens injections
the hypothalamus limits accumbens dopamine release while increasing
produce a place preference that is blocked by cis-flupenthixol, Pharmacol.
acetylcholine: a possible satiation mechanism, Brain Res. 963 (2003) 290–
Biochem. Behav. 46 (1993) 543–552.
[187] J.H. Kagel, R.C. Battalio, L. Green, Economic Choice Theory. An Experimental
[158] L. Hernandez, B.G. Hoebel, Food reward and cocaine increase extracellular
Analysis of Animal Behavior, Cambridge University Press, 1995.
dopamine in the nucleus accumbens as measured by microdialysis, Life Sci.
[188] P.W. Kalivas, J. Stewart, Dopamine transmission in the initiation and
42 (1988) 1705–1712.
expression of drug- and stress-induced sensitization of motor activity,
[159] R.J. Herrnstein, Formal properties of the matching law, J. Exp. Anal. Behav. 21
Brain Res. Brain Res. Rev. 16 (1991) 223–244.
(1974) 159–164.
[189] A.E. Kelley, B.A. Baldo, W.E. Pratt, M.J. Will, Corticostriatal-hypothalamic
[160] S. Higgs, D.J. Barber, A.J. Cooper, P. Terry, Differential effects of two
circuitry and food motivation: integration of energy, action and reward,
cannabinoid receptor agonists on progressive ratio responding for food and
Physiol. Behav. 86 (2005) 773–795.
free-feeding in rats, Behav. Pharmacol. 16 (2005) 389–393.
[190] A.E. Kelley, M. Cador, L. Stinus, M. Le Moal, Neurotensin, substance P,
[161] S. Higgs, C.M. Williams, T.C. Kirkham, Cannabinoid influences on palatability:
neurokinin-alpha, and enkephalin: injection into ventral tegmental area in
tetrahydrocannabinol, anandamide, 2-arachidonoyl glycerol and SR141716,
Psychopharmacology (Berl.) 97 (1989) 243–252.
Psychopharmacology (Berl.) 165 (2003) 370–377.
[191] A.E. Kelley, J.M. Delfs, Dopamine and conditioned reinforcement. I.
[162] W. Hodos, Progressive ratio as a measure of reward strength, Science 134
Differential effects of amphetamine microinjections into striatal subregions,
(1961) 943–944.
Psychopharmacology (Berl.) 103 (1991) 187–196.
[163] W. Hodos, G. Kalman, Effects of increment size and reinforcer volume on
[192] A.E. Kelley, J.M. Delfs, Dopamine and conditioned reinforcement. II.
progressive ratio performance, J. Exp. Anal. Behav. 6 (1963) 387–392.
Contrasting effects of amphetamine microinjection into the nucleus
[164] B.G. Hoebel, Inhibition and disinhibition of self-stimulation and feeding:
accumbens with peptide microinjection into the ventral tegmental area,
hypothalamic contol and postingestional factors, J. Comp. Physiol. Psychol. 66
Psychopharmacology (Berl.) 103 (1991) 197–203.
(1968) 89–100.
[193] Y. Kitabatake, T. Hikida, D. Watanabe, I. Pastan, S. Nakanishi, Impairment of
[165] B.G. Hoebel, N.M. Avena, P. Rada, Accumbens dopamine–acetylcholine
reward-related learning by cholinergic cell ablation in the striatum, Proc.
balance in approach and avoidance, Curr. Opin. Pharmacol. 7 (2007) 617–
Natl. Acad. Sci. USA 100 (2003) 7965–7970.
[194] L. Kokkinidis, B.D. McCarter, Postcocaine depression and sensitization of
[166] B.G. Hoebel, A.P. Monaco, L. Hernandez, E.F. Aulisi, B.G. Stanley, L. Lenard,
brain-stimulation reward: analysis of reinforcement and performance effects,
Self-injection of amphetamine directly into the brain, Psychopharmacology
Pharmacol. Biochem. Behav. 36 (1990) 463–471.
(Berl.) 81 (1983) 158–163.
[195] M.G. Kolta, P. Shreve, N.J. Uretsky, Effect of pretreatment with amphetamine
[167] B.G. Hoebel, P. Teitelbaum, Hypothalamic control of feeding and self-
on the interaction between amphetamine and dopamine neurons in the
stimulation, Science 135 (1962) 375–377.
nucleus accumbens, Neuropharmacology 28 (1989) 9–14.
[168] B.G. Hoebel, R.D. Thompson, Aversion to lateral hypothalamic stimulation
[196] G.F. Koob, S.J. Riley, S.C. Smith, T.W. Robbins, Effects of 6-hydroxydopamine
caused by intragastric feeding or obesity, J. Comp. Physiol. Psychol. 68 (1969)
lesions of the nucleus accumbens septi and olfactory tubercle on feeding,
locomotor activity, and amphetamine anorexia in the rat, J. Comp. Physiol.
[169] P.C. Holland, G.D. Petrovich, A neural systems analysis of the potentiation of
Psychol. 92 (1978) 917–927.
feeding by conditioned stimuli, Physiol. Behav. 86 (2005) 747–761.
[197] A.C. Kreitzer, R.C. Malenka, Striatal plasticity and basal ganglia circuit
[170] J.D. Hommel, R. Trinko, R.M. Sears, D. Georgescu, Z.W. Liu, X.B. Gao, J.J.
function, Neuron 60 (2008) 543–554.
Thurmon, M. Marinelli, R.J. DiLeone, Leptin receptor signaling in midbrain
[198] U. Krugel, T. Schraft, H. Kittner, W. Kiess, P. Illes, Basal and feeding-evoked
dopamine neurons regulates feeding, Neuron 51 (2006) 801–810.
dopamine release in the rat nucleus accumbens is depressed by leptin, Eur. J.
[171] G.W. Hubert, M.J. Kuhar, Colocalization of CART peptide with prodynorphin
Pharmacol. 482 (2003) 185–187.
and dopamine D1 receptors in the rat nucleus accumbens, Neuropeptides 40
[199] S.A. Kushner, S.L. Dewey, C. Kornetsky, The irreversible gamma-aminobutyric
(2006) 409–415.
acid (GABA) transaminase inhibitor gamma-vinyl-GABA blocks cocaine self-
[172] Y.L. Hurd, F. Weiss, G.F. Koob, N.E. And, U. Ungerstedt, Cocaine reinforcement
administration in rats, J. Pharmacol. Exp. Ther. 290 (1999) 797–802.
and extracellular dopamine overflow in rat nucleus accumbens: an in vivo
[200] S.E. la Fleur, L.J. Vanderschuren, M.C. Luijendijk, B.M. Kloeze, B. Tiesjema, R.A.
microdialysis study, Brain Res. 498 (1989) 199–203.
Adan, A reciprocal interaction between food-motivated behavior and diet-
[173] S.R. Hursh, Behavioral economics, J. Exp. Anal. Behav. 42 (1984) 435–452.
induced obesity, Int. J. Obes. (London) 31 (2007) 1286–1294.
S. Fulton / Frontiers in Neuroendocrinology 31 (2010) 85–103
[201] D.D. Lam, L.K. Heisler, Serotonin and energy balance. molecular mechanisms
[229] G.J. Mogenson, D.L. Jones, C.Y. Yim, From motivation to action: functional
and implications for type 2 diabetes, Expert Rev. Mol. Med. 9 (2007) 1–24.
interface between the limbic system and the motor system, Prog. Neurobiol.
[202] B. Le Foll, C.E. Wertheim, S.R. Goldberg, Effects of baclofen on conditioned
14 (1980) 69–97.
rewarding and discriminative stimulus effects of nicotine in rats, Neurosci.
[230] R.F. Mucha, S.D. Iversen, Increased food intake after opioid microinjections
Lett. 443 (2008) 236–240.
into nucleus accumbens and ventral tegmental area of rat, Brain Res. 397
[203] G.M. Leinninger, Y.H. Jo, R.L. Leshan, G.W. Louis, H. Yang, J.G. Barrera, H.
(1986) 214–224.
Wilson, D.M. Opland, M.A. Faouzi, Y. Gong, J.C. Jones, C.J. Rhodes, S. Chua Jr., S.
[231] M.G. Myers, M.A. Cowley, H. Munzberg, Mechanisms of leptin action and
Diano, T.L. Horvath, R.J. Seeley, J.B. Becker, H. Munzberg, M.G. Myers Jr.,
leptin resistance, Annu. Rev. Physiol. 70 (2008) 537–556.
Leptin acts via leptin receptor-expressing lateral hypothalamic neurons to
[232] M.G. Myers Jr., H. Munzberg, G.M. Leinninger, R.L. Leshan, The geometry of
modulate the mesolimbic dopamine system and suppress feeding, Cell
leptin action in the brain: more complicated than a simple ARC, Cell Metab. 9
Metab. 10 (2009) 89–98.
(2009) 117–123.
[204] A.S. Lippa, S.M. Antelman, A.E. Fisher, D.R. Canfield, Neurochemical mediation
[233] M.A. Nader, J.E. Barrett, Effects of corticotropin-releasing factor (CRF), tuftsin
of reward: a significant role for dopamine?, Pharmacol Biochem. Behav. 1
and dermorphin on behavior of squirrel monkeys maintained by different
(1973) 23–28.
events, Peptides 10 (1989) 1199–1204.
[205] D.S. Lorrain, G.M. Arnold, P. Vezina, Previous exposure to amphetamine
[234] S.G. Nair, S.A. Golden, Y. Shaham, Differential effects of the hypocretin 1
increases incentive to obtain the drug: long-lasting effects revealed by the
receptor antagonist SB 334867 on high-fat food self-administration and
progressive ratio schedule, Behav. Brain Res. 107 (2000) 9–19.
reinstatement of food seeking in rats, Br. J. Pharmacol. 154 (2008) 406–416.
[206] B.J. Lute, H. Khoshbouei, C. Saunders, N. Sen, R.Z. Lin, J.A. Javitch, A. Galli, PI3K
[235] A.M. Naleid, M.K. Grace, M. Chimukangara, C.J. Billington, A.S. Levine,
Paraventricular opioids alter intake of high-fat but not high-sucrose diet
Biophys. Res. Commun. 372 (2008) 656–661.
depending on diet preference in a binge model of feeding, Am. J. Physiol.
[207] W.C. Lynch, L. Libby, Naloxone suppresses intake of highly preferred
Regul. Integr. Comp. Physiol. 293 (2007) R99–R105.
saccharin solutions in food deprived and sated rats, Life Sci. 33 (1983)
[236] A.M. Naleid, M.K. Grace, D.E. Cummings, A.S. Levine, Ghrelin induces feeding
in the mesolimbic reward pathway between the ventral tegmental area and
[208] W.H. Lyness, N.M. Friedle, K.E. Moore, Destruction of dopaminergic nerve
the nucleus accumbens, Peptides 26 (2005) 2274–2279.
[237] P. Nencini, J. Stewart, Chronic systemic administration of amphetamine
administration, Pharmacol. Biochem. Behav. 11 (1979) 553–556.
increases food intake to morphine, but not to U50–488H, microinjected into
[209] P. Maccioni, D. Pes, M.A. Carai, G.L. Gessa, G. Colombo, Suppression by the
the ventral tegmental area in rats, Brain Res. 527 (1990) 254–258.
cannabinoid CB1 receptor antagonist, rimonabant, of the reinforcing and
[238] R. Nieuwenhuys, L.M. Geeraedts, J.G. Veening, The medial forebrain bundle of
motivational properties of a chocolate-flavoured beverage in rats, Behav.
the rat. I. General introduction, J. Comp. Neurol. 206 (1982) 49–81.
Pharmacol. 19 (2008) 197–209.
[239] M.B. Noel, R.A. Wise, Ventral tegmental injections of morphine but not U-50,
[210] M.J. Macenski, D.W. Schaal, J. Cleary, T. Thompson, Changes in food-
488H enhance feeding in food-deprived rats, Brain Res. 632 (1993) 68–73.
[240] R. Norgren, Gustatory responses in the hypothalamus, Brain Res. 21 (1970)
buprenorphine or methadone administration, Pharmacol. Biochem. Behav.
47 (1994) 379–383.
[241] R. Norgren, C.M. Leonard, Ascending central gustatory pathways, J. Comp.
[211] S.V. Mahler, K.S. Smith, K.C. Berridge, Endocannabinoid hedonic hotspot for
Neurol. 150 (1973) 217–237.
sensory pleasure: anandamide in nucleus accumbens shell enhances ‘liking'
[242] H. Ogawa, Gustatory cortex of primates: anatomy and physiology, Neurosci.
of a sweet reward, Neuropsychopharmacology 32 (2007) 2267–2278.
Res. 20 (1994) 1–13.
[212] N.H. Majeed, B. Przewlocka, K. Wedzony, R. Przewlocki, Stimulation of food
[243] J. Olds, Satiation effects in self-stimulation of the brain, J. Comp. Physiol.
intake following opioid microinjection into the nucleus accumbens septi in
Psychol. 51 (1958) 675–678.
rats, Peptides 7 (1986) 711–716.
[244] J. Olds, Self-stimulation of the brain; its use to study local effects of hunger,
[213] C.S. Maldonado-Irizarry, C.J. Swanson, A.E. Kelley, Glutamate receptors in the
sex, and drugs, Science 127 (1958) 315–324.
[245] J. Olds, P.M. Milner, Positive reinforcement produced by electrical stimulation
hypothalamus, J. Neurosci. 15 (1995) 6779–6788.
of septal area and other regions of rat brain, J. Comp. Physiol. Psychol. 47
[214] S. Malik, F. McGlone, D. Bedrossian, A. Dagher, Ghrelin modulates brain
(1954) 419–427.
activity in areas that control appetitive behavior, Cell Metab. 7 (2008) 400–
[246] J. Olds, M.E. Olds, The mechanisms of voluntary behavior, in: R.G. Heath (Ed.),
The Role of Pleasure in Behavior, Harper and Row, New York, 1964, pp. 23–
[215] D.L. Margules, J. Olds, Identical ‘‘feeding" and ‘‘rewarding" systems in the
lateral hypothalamus of rats, Science 135 (1962) 374–375.
[247] M.E. Olds, Reinforcing effects of morphine in the nucleus accumbens, Brain
[216] G.P. Mark, A.E. Kinney, M.C. Grubb, X. Zhu, D.A. Finn, S.L. Mader, S.P. Berger,
Res. 237 (1982) 429–440.
A.J. Bechtholt, Injection of oxotremorine in nucleus accumbens shell reduces
[248] Y. Oomura, Glucose as a regulator of neuronal activity, in: A.J. Szabo (Ed.),
cocaine but not food self-administration in rats, Brain Res. 1123 (2006) 51–
Advances in Metabolic Disorders, Academic, New York, 1983, pp. 31–65.
[249] R.D. Palmiter, Dopamine signaling in the dorsal striatum is essential for
[217] G.P. Mark, P. Rada, E. Pothos, B.G. Hoebel, Effects of feeding and drinking on
motivated behaviors: lessons from dopamine-deficient mice, Ann. N.Y. Acad.
acetylcholine release in the nucleus accumbens, striatum, and hippocampus
Sci. 1129 (2008) 35–46.
of freely behaving rats, J. Neurochem. 58 (1992) 2269–2274.
[250] R.F. Parrott, Central administration of corticotropin releasing factor in the
[218] A. Markou, N.E. Paterson, S. Semenova, Role of gamma-aminobutyric acid
pig: effects on operant feeding, drinking and plasma cortisol, Physiol. Behav.
(GABA) and metabotropic glutamate receptors in nicotine reinforcement:
47 (1990) 519–524.
potential pharmacotherapies for smoking cessation, Ann. N.Y. Acad. Sci. 1025
[251] N.E. Paterson, W. Froestl, A. Markou, The GABAB receptor agonists baclofen
(2004) 491–503.
[219] P. Martel, M. Fantino, Influence of the amount of food ingested on mesolimbic
Psychopharmacology (Berl.) 172 (2004) 179–186.
dopaminergic system activity: a microdialysis study, Pharmacol. Biochem.
[252] N.E. Paterson, A. Markou, The metabotropic glutamate receptor 5 antagonist
Behav. 55 (1996) 297–302.
MPEP decreased break points for nicotine, cocaine and food in rats,
[220] R.D. Mattes, Orosensory considerations, Obesity (Silver Spring) 14 (Suppl. 4)
Psychopharmacology (Berl.) 179 (2005) 255–261.
(2006) 164S–167S.
[253] S. Pecina, K.C. Berridge, Opioid site in nucleus accumbens shell mediates
[221] M.L. McCaleb, R.D. Myers, Striatal dopamine release is altered by glucose and
eating and hedonic ‘liking' for food: map based on microinjection Fos plumes,
insulin during push–pull perfusion of the rat's caudate nucleus, Brain Res.
Brain Res. 863 (2000) 71–86.
Bull. 4 (1979) 651–656.
[254] S. Pecina, K.C. Berridge, L.A. Parker, Pimozide does not shift palatability:
[222] S.A. McCaughey, The taste of sugars, Neurosci. Biobehav. Rev. 32 (2008)
separation of anhedonia from sensorimotor suppression by taste reactivity,
Pharmacol. Biochem. Behav. 58 (1997) 801–811.
[223] A. McGregor, D.C. Roberts, Dopaminergic antagonism within the nucleus
[255] A.G. Phillips, S. Ahn, S.B. Floresco, Magnitude of dopamine release in medial
accumbens or the amygdala produces differential effects on intravenous
prefrontal cortex predicts accuracy of memory on a delayed response task, J.
cocaine self-administration under fixed and progressive ratio schedules of
Neurosci. 24 (2004) 547–553.
reinforcement, Brain Res. 624 (1993) 245–252.
[256] A.G. Phillips, C.D. Blaha, H.C. Fibiger, Neurochemical correlates of brain-
[224] E. Milliaresis, P. Rompre, P. Laviolette, L. Philippe, D. Coulombe, The curve-
stimulation reward measured by ex vivo and in vivo analyses, Neurosci.
shift paradigm in self-stimulation, Physiol. Behav. 37 (1986) 85–91.
Biobehav. Rev. 13 (1989) 99–104.
[225] P.M. Milner, The discovery of self-stimulation and other stories, Neurosci.
[257] A.G. Phillips, G. Vacca, S. Ahn, A top-down perspective on dopamine,
Biobehav. Rev. 13 (1989) 61–67.
motivation and memory, Pharmacol. Biochem. Behav. 90 (2008) 236–249.
[226] J.B. Mitchell, A. Gratton, Partial dopamine depletion of the prefrontal cortex
[258] P.V. Piazza, J.M. Deminiere, M. Le Moal, H. Simon, Factors that predict
leads to enhanced mesolimbic dopamine release elicited by repeated
individual vulnerability to amphetamine self-administration, Science 245
exposure to naturally reinforcing stimuli, J. Neurosci. 12 (1992) 3609–3618.
(1989) 1511–1513.
[227] M. Miura, M. Masuda, T. Aosaki, Roles of micro-opioid receptors in GABAergic
[259] V.M. Pickel, J. Chan, T.L. Kash, J.J. Rodriguez, K. MacKie, Compartment-specific
synaptic transmission in the striosome and matrix compartments of the
localization of cannabinoid 1 (CB1) and mu-opioid receptors in rat nucleus
striatum, Mol. Neurobiol. 37 (2008) 104–115.
accumbens, Neuroscience 127 (2004) 101–112.
[228] G. Miyata, M.M. Meguid, S.O. Fetissov, G.F. Torelli, H.J. Kim, Nicotine's effect
[260] C. Pickering, J. Alsio, A.L. Hulting, H.B. Schioth, Withdrawal from free-choice
on hypothalamic neurotransmitters and appetite regulation, Surgery 126
high-fat high-sugar diet induces craving only in obesity-prone animals,
(1999) 255–263.
Psychopharmacology (Berl.) (2009).
S. Fulton / Frontiers in Neuroendocrinology 31 (2010) 85–103
[261] P. Pissios, L. Frank, A.R. Kennedy, D.R. Porter, F.E. Marino, F.F. Liu, E.N. Pothos,
[291] J.M. Rudski, C.J. Billington, A.S. Levine, Naloxone's effects on operant
E. Maratos-Flier, Dysregulation of the mesolimbic dopamine system and
responding depend upon level of deprivation, Pharmacol. Biochem. Behav.
mice, Biol. Psychiat. 64 (2008) 184–191.
49 (1994) 377–383.
[262] W.E. Pratt, K. Blackstone, Nucleus accumbens acetylcholine and food intake:
[292] S.J. Russo, C.A. Bolanos, D.E. Theobald, N.A. DeCarolis, W. Renthal, A. Kumar,
decreased muscarinic tone reduces feeding but not food-seeking, Behav.
C.A. Winstanley, N.E. Renthal, M.D. Wiley, D.W. Self, D.S. Russell, R.L. Neve,
Brain Res. 198 (2009) 252–257.
A.J. Eisch, E.J. Nestler, IRS2-Akt pathway in midbrain dopamine neurons
[263] W.E. Pratt, A.E. Kelley, Nucleus accumbens acetylcholine regulates appetitive
regulates behavioral and cellular responses to opiates, Nat. Neurosci. 10
learning and motivation for food via activation of muscarinic receptors,
(2007) 93–99.
Behav. Neurosci. 118 (2004) 730–739.
[293] Y. Saito, M. Cheng, F.M. Leslie, O. Civelli, Expression of the melanin-
[264] W.E. Pratt, A.E. Kelley, Striatal muscarinic receptor antagonism reduces 24-h
concentrating hormone (MCH) receptor mRNA in the rat brain, J. Comp.
food intake in association with decreased preproenkephalin gene expression,
Neurol. 435 (2001) 26–40.
Eur. J. Neurosci. 22 (2005) 3229–3240.
[294] J.D. Salamone, M. Correa, A. Farrar, S.M. Mingote, Effort-related functions of
[265] D. Quarta, C. Di Francesco, S. Melotto, L. Mangiarini, C. Heidbreder, G. Hedou,
Systemic administration of ghrelin increases extracellular dopamine in the
Psychopharmacology (Berl.) 191 (2007) 461–482.
shell but not the core subdivision of the nucleus accumbens, Neurochem. Int.
[295] J.D. Salamone, R.E. Steinpreis, L.D. McCullough, P. Smith, D. Grebel, K. Mahan,
54 (2009) 89–94.
Haloperidol and nucleus accumbens dopamine depletion suppress lever
[266] P.V. Rada, G.P. Mark, J.J. Yeomans, B.G. Hoebel, Acetylcholine release in
pressing for food but increase free food consumption in a novel food choice
ventral tegmental area by hypothalamic self-stimulation, eating, and
procedure, Psychopharmacology (Berl.) 104 (1991) 515–521.
drinking, Pharmacol. Biochem. Behav. 65 (2000) 375–379.
[296] P. Samama, L. Rumennik, J.F. Grippo, The melanocortin receptor MCR4
[267] E.B. Rasmussen, S.L. Huskinson, Effects of rimonabant on behavior
controls fat consumption, Regul. Pept. 113 (2003) 85–88.
maintained by progressive ratio schedules of sucrose reinforcement in
[297] C. Sanchis-Segura, B.H. Cline, G. Marsicano, B. Lutz, R. Spanagel, Reduced
obese Zucker (fa/fa) rats, Behav. Pharmacol. 19 (2008) 735–742.
sensitivity to reward in CB1 knockout mice, Psychopharmacology (Berl.) 176
[268] P. Redgrave, K. Gurney, The short-latency dopamine signal: a role in
(2004) 223–232.
discovering novel actions?, Nat Rev. Neurosci. 7 (2006) 967–975.
[298] A.C. Sanders, A.J. Hussain, R. Hen, X. Zhuang, Chronic blockade or constitutive
[269] S. Reilly, Reinforcement value of gustatory stimuli determined by progressive
deletion of the serotonin transporter reduces operant responding for food
ratio performance, Pharmacol. Biochem. Behav. 63 (1999) 301–311.
reward, Neuropsychopharmacology 32 (2007) 2321–2329.
[270] J.K. Richards, J.A. Simms, P. Steensland, S.A. Taha, S.L. Borgland, A. Bonci, S.E.
[299] D.J. Sanger, P.S. McCarthy, Differential effects of morphine on food and water
Bartlett, Inhibition of orexin-1/hypocretin-1 receptors inhibits yohimbine-
intake in food deprived and freely-feeding rats, Psychopharmacology (Berl.)
induced reinstatement of ethanol and sucrose seeking in Long-Evans rats,
72 (1980) 103–106.
Psychopharmacology (Berl.) 199 (2008) 109–117.
[300] C.B. Saper, L.W. Swanson, W.M. Cowan, An autoradiographic study of the
[271] N.R. Richardson, A. Gratton, Changes in medial prefrontal cortical dopamine
efferent connections of the lateral hypothalamic area in the rat, J. Comp.
levels associated with response-contingent food reward: an electrochemical
Neurol. 183 (1979) 689–706.
study in rat, J. Neurosci. 18 (1998) 9130–9138.
[301] A.N. Schoffelmeer, F. Hogenboom, G. Wardeh, T.J. De Vries, Interactions
[272] D.C. Roberts, M.M. Andrews, G.J. Vickers, Baclofen attenuates the reinforcing
between CB1 cannabinoid and mu opioid receptors mediating inhibition of
effects of cocaine in rats, Neuropsychopharmacology 15 (1996) 417–423.
neurotransmitter release in rat nucleus accumbens core, Neuropharmacology
[273] D.C. Roberts, M.E. Corcoran, H.C. Fibiger, On the role of ascending
51 (2006) 773–781.
catecholaminergic systems in intravenous self-administration of cocaine,
[302] W. Schultz, Behavioral dopamine signals, Trends Neurosci. 30 (2007) 203–
Pharmacol. Biochem. Behav. 6 (1977) 615–620.
[274] D.L. Robinson, B.J. Venton, M.L. Heien, R.M. Wightman, Detecting subsecond
[303] W. Schultz, P. Dayan, P.R. Montague, A neural substrate of prediction and
dopamine release with fast-scan cyclic voltammetry in vivo, Clin. Chem. 49
reward, Science 275 (1997) 1593–1599.
(2003) 1763–1773.
[304] A. Sclafani, Post-ingestive positive controls of ingestive behavior, Appetite 36
[275] S. Robinson, A.J. Rainwater, T.S. Hnasko, R.D. Palmiter, Viral restoration of
(2001) 79–83.
dopamine signaling to the dorsal striatum restores instrumental conditioning
[305] A. Sclafani, Psychobiology of food preferences, Int. J. Obes. Relat. Metab.
to dopamine-deficient mice, Psychopharmacology (Berl.) 191 (2007) 567–
Disord. 25 (Suppl. 5) (2001) S13–S16.
[306] A. Sclafani, K. Ackroff, Deprivation alters rats' flavor preferences for
[276] S. Robinson, S.M. Sandstrom, V.H. Denenberg, R.D. Palmiter, Distinguishing
carbohydrates and fats, Physiol. Behav. 53 (1993) 1091–1099.
whether dopamine regulates liking, wanting, and/or learning about rewards,
[307] J.W. Scott, C. Pfaffmann, Characteristics of responses of lateral hypothalamic
Behav. Neurosci. 119 (2005) 5–15.
neurons to stimulation of the olfactory system, Brain Res. 48 (1972) 251–264.
[277] T.E. Robinson, B. Kolb, Alterations in the morphology of dendrites and
[308] M.M. Scott, J.L. Lachey, S.M. Sternson, C.E. Lee, C.F. Elias, J.M. Friedman, J.K.
dendritic spines in the nucleus accumbens and prefrontal cortex following
Elmquist, Leptin targets in the mouse brain, J. Comp. Neurol. 514 (2009) 518–
repeated treatment with amphetamine or cocaine, Eur. J. Neurosci. 11 (1999)
[309] M.A. Segall, D.L. Margules, Central mediation of naloxone-induced anorexia
[278] T.E. Robinson, B. Kolb, Structural plasticity associated with exposure to drugs
in the ventral tegmental area, Behav. Neurosci. 103 (1989) 857–864.
of abuse, Neuropharmacology 47 (Suppl. 1) (2004) 33–46.
[310] U. Shalev, J. Yap, Y. Shaham, Leptin attenuates acute food deprivation-
[279] G.H. Rogers, H. Frenk, A.N. Taylor, J.C. Liebeskind, Naloxone suppression of
induced relapse to heroin seeking, J. Neurosci. 21 (2001) RC129.
food and water intake in deprived rats, Proc. West. Pharmacol. Soc. 21 (1978)
[311] C.J. Shi, M.D. Cassell, Cortical, thalamic, and amygdaloid connections of the
anterior and posterior insular cortices, J. Comp. Neurol. 399 (1998) 440–
[280] G. Rogge, D. Jones, G.W. Hubert, Y. Lin, M.J. Kuhar, CART peptides: regulators
of body weight, reward and other functions, Nat. Rev. Neurosci. 9 (2008)
[312] P. Shizgal, Toward a cellular analysis of intracranial self-stimulation:
contributions of collision studies, Neurosci. Biobehav. Rev. 13 (1989) 81–90.
[281] M.F. Roitman, G.D. Stuber, P.E. Phillips, R.M. Wightman, R.M. Carelli,
[313] P. Shizgal, Neural basis of utility estimation, Curr. Opin. Neurobiol. 7 (1997)
Dopamine operates as a subsecond modulator of food seeking, J. Neurosci.
24 (2004) 1265–1271.
[314] P. Shizgal, On the neural computation of utility: implications from studies of
[282] M.F. Roitman, R.A. Wheeler, R.M. Carelli, Nucleus accumbens neurons are
brain stimulation reward, in: E.D.N.S.D. Kahneman (Ed.), Well-Being: The
innately tuned for rewarding and aversive taste stimuli, encode their
Foundations of Hedonic Psychology, Russell Sage Foundation, New York,
predictors, and are linked to motor output, Neuron 45 (2005) 587–597.
1999, pp. 502–526.
[283] M.F. Roitman, R.A. Wheeler, R.M. Wightman, R.M. Carelli, Real-time chemical
[315] P. Shizgal, C. Bielajew, D. Corbett, R. Skelton, J. Yeomans, Behavioral methods
responses in the nucleus accumbens differentiate rewarding and aversive
for inferring anatomical linkage between rewarding brain stimulation sites, J.
stimuli, Nat. Neurosci. 11 (2008) 1376–1377.
Comp. Physiol. Psychol. 94 (1980) 227–237.
[284] E. Rolls, M. Burton, F. Mora, Neurophyiological analysis of brain-stimulation
[316] P. Shizgal, S. Fulton, B. Woodside, Brain reward circuitry and the regulation of
reward in the monkey, Brain Res. 194 (1980) 339–357.
energy balance, Int. J. Obes. Relat. Metab. Disord. 25 (Suppl. 5) (2001) S17–
[285] E.T. Rolls, M.J. Burton, F. Mora, Hypothalamic neuronal responses associated
with the sight of food, Brain Res. 111 (1976) 53–66.
[317] S.A. Simon, I.E. de Araujo, R. Gutierrez, M.A. Nicolelis, The neural mechanisms
[286] P.P. Rompre, R.A. Wise, Opioid–neuroleptic interaction in brainstem self-
of gustation: a distributed processing code, Nat. Rev. Neurosci. 7 (2006) 890–
stimulation, Brain Res. 477 (1989) 144–151.
[287] A.G. Roseberry, T. Painter, G.P. Mark, J.T. Williams, Decreased vesicular
[318] B.F. Skinner, The Behavior of Organisms: An Experimental Analysis,
somatodendritic dopamine stores in leptin-deficient mice, J. Neurosci. 27
Appelton-Century, New York, 1938.
(2007) 7021–7027.
[319] S.L. Smith-Roe, A.E. Kelley, Coincident activation of NMDA and dopamine D1
[288] E. Rossitch Jr., R.A. King, D. Luttinger, C.B. Nemeroff, Behavioral effects of
receptors within the nucleus accumbens core is required for appetitive
neurotensin: operant responding and assessment of ‘anhedonia', Eur. J.
instrumental learning, J. Neurosci. 20 (2000) 7737–7742.
Pharmacol. 163 (1989) 119–122.
[320] K.S. Smith, K.C. Berridge, The ventral pallidum and hedonic reward:
[289] A. Routtenberg, J. Lindy, Effects of the availability of rewarding septal and
neurochemical maps of sucrose ‘‘liking" and food intake, J. Neurosci. 25
hypothalamic stimulation on bar pressing for food under conditions of
(2005) 8637–8649.
deprivation, J. Comp. Physiol. Psychol. 60 (1965) 158–161.
[321] J.D. Sokolowski, A.N. Conlan, J.D. Salamone, A microdialysis study of nucleus
[290] P. Rozin, Acquisition of stable food preferences, Nutr. Rev. 48 (1990) 106–113
accumbens core and shell dopamine during operant responding in the rat,
Neuroscience 86 (1998) 1001–1009.
S. Fulton / Frontiers in Neuroendocrinology 31 (2010) 85–103
[322] M. Solinas, S.R. Goldberg, Motivational effects of cannabinoids and opioids on
[350] L.A. Velloso, E.P. Araujo, C.T. de Souza, Diet-induced inflammation of the
food reinforcement depend on simultaneous activation of cannabinoid and
hypothalamus in obesity, Neuroimmunomodulation 15 (2008) 189–193.
opioid systems, Neuropsychopharmacology 30 (2005) 2035–2045.
[351] P. Vezina, Sensitization of midbrain dopamine neuron reactivity and the self-
[323] L.A. Sombers, M. Beyene, R.M. Carelli, R.M. Wightman, Synaptic overflow of
administration of psychomotor stimulant drugs, Neurosci. Biobehav. Rev. 27
dopamine in the nucleus accumbens arises from neuronal activity in the
(2004) 827–839.
ventral tegmental area, J. Neurosci. 29 (2009) 1735–1742.
[352] N.D. Volkow, G.J. Wang, F. Telang, J.S. Fowler, P.K. Thanos, J. Logan, D. Alexoff,
[324] G. Soria, V. Mendizabal, C. Tourino, P. Robledo, C. Ledent, M. Parmentier, R.
Y.S. Ding, C. Wong, Y. Ma, K. Pradhan, Low dopamine striatal D2 receptors are
Maldonado, O. Valverde, Lack of CB1 cannabinoid receptor impairs cocaine
self-administration, Neuropsychopharmacology 30 (2005) 1670–1680.
contributing factors, Neuroimage 42 (2008) 1537–1543.
[325] G. Spies, Food versus intracranial self-stimulation reinforcement in food-
[353] G.J. Wang, N.D. Volkow, J. Logan, N.R. Pappas, C.T. Wong, W. Zhu, N. Netusil,
deprived rats, J. Comp. Physiol. Psychol. 60 (1965) 153–157.
J.S. Fowler, Brain dopamine and obesity, Lancet 357 (2001) 354–357.
[326] C. Spyraki, H.C. Fibiger, A.G. Phillips, Attenuation by haloperidol of place
[354] S.J. Ward, L.A. Dykstra, The role of CB1 receptors in sweet versus fat
preference conditioning using food reinforcement, Psychopharmacology
reinforcement: effect of CB1 receptor deletion, CB1 receptor antagonism
(Berl.) 77 (1982) 379–382.
(SR141716A) and CB1 receptor agonism (CP-55940), Behav. Pharmacol. 16
[327] D.N. Stephens, G. Brown, Disruption of operant oral self-administration of
(2005) 381–388.
ethanol, sucrose, and saccharin by the AMPA/kainate antagonist, NBQX, but
[355] P.J. Wellman, K.W. Davis, J.R. Nation, Augmentation of cocaine hyperactivity
not the AMPA antagonist, GYKI 52466, Alcohol Clin. Exp. Res. 23 (1999)
in rats by systemic ghrelin, Regul. Pept. 125 (2005) 151–154.
[356] M.J. Will, E.B. Franzblau, A.E. Kelley, Nucleus accumbens mu-opioids regulate
[328] E. Stice, S. Spoor, C. Bohon, D.M. Small, Relation between obesity and blunted
intake of a high-fat diet via activation of a distributed brain network, J.
striatal response to food is moderated by TaqIA A1 allele, Science 322 (2008)
Neurosci. 23 (2003) 2882–2888.
[357] C. Wilson, G.G. Nomikos, M. Collu, H.C. Fibiger, Dopaminergic correlates of
[329] T.R. Stratford, C.J. Swanson, A. Kelley, Specific changes in food intake elicited
motivated behavior: importance of drive, J. Neurosci. 15 (1995) 5169–
by blockade or activation of glutamate receptors in the nucleus accumbens
shell, Behav. Brain Res. 93 (1998) 43–50.
[330] D.J. Surmeier, J. Ding, M. Day, Z. Wang, W. Shen, D1 and D2 dopamine-
receptor modulation of striatal glutamatergic signaling in striatal medium
stimulation of the substantia nigra in the rat, Behav. Neurosci. 97 (1983)
spiny neurons, Trends Neurosci. 30 (2007) 228–235.
[331] L.W. Swanson, Cerebral hemisphere regulation of motivated behavior, Brain
[359] R.A. Wise, Forebrain substrates of reward and motivation, J. Comp. Neurol.
Res. 886 (2000) 113–164.
493 (2005) 115–121.
[332] S.L. Teegarden, E.J. Nestler, T.L. Bale, Delta FosB-mediated alterations in
[360] F.H. Wojnicki, D.C. Roberts, R.L. Corwin, Effects of baclofen on operant
dopamine signaling are normalized by a palatable high-fat diet, Biol.
performance for food pellets and vegetable shortening after a history of
Psychiat. 64 (2008) 941–950.
binge-type behavior in non-food deprived rats, Pharmacol. Biochem. Behav.
[333] J.L. Temple, C.M. Legierski, A.M. Giacomelli, S.J. Salvy, L.H. Epstein,
84 (2006) 197–206.
Overweight children find food more reinforcing and consume more energy
than do nonoverweight children, Am. J. Clin. Nutr. 87 (2008) 1121–1127.
intracerebroventricular infusion of insulin reduces food intake and body
[334] D.A. Thompson, R.G. Campbell, Hunger in humans induced by 2-deoxy-D-
weight of baboons, Nature 282 (1979) 503–505.
glucose: glucoprivic control of taste preference and food intake, Science 198
[362] C.L. Wyvell, K.C. Berridge, Intra-accumbens amphetamine increases the
(1977) 1065–1068.
conditioned incentive salience of sucrose reward: enhancement of reward
[335] E.L. Thorndike, Animal Intelligence: An experimental study of the associative
‘‘wanting" without enhanced ‘‘liking" or response reinforcement, J. Neurosci.
processes in animalsPsychological Review, Monograph Supplements, vol. 8,
20 (2000) 8122–8130.
MacMillan, New york, 1898.
[363] J.S. Yeomans, Two substrates for medial forebrain bundle self-stimulation:
[336] Z.D. Thornton-Jones, S.P. Vickers, P.G. Clifton, The cannabinoid CB1 receptor
myelinated axons and dopamine axons, Neurosci. Biobehav. Rev. 13 (1989)
antagonist SR141716A reduces appetitive and consummatory responses for
food, Psychopharmacology (Berl.) 179 (2005) 452–460.
[364] J.S. Yeomans, N.T. Maidment, B.S. Bunney, Excitability properties of medial
[337] A.J. Thorpe, J.P. Cleary, A.S. Levine, C.M. Kotz, Centrally administered orexin A
forebrain bundle axons of A9 and A10 dopamine cells, Brain Res. 450 (1988)
increases motivation for sweet pellets in rats, Psychopharmacology (Berl.)
182 (2005) 75–83.
[365] J.S. Yeomans, A. Mathur, M. Tampakeras, Rewarding brain stimulation: role of
[338] A.J. Thorpe, M.A. Mullett, C. Wang, C.M. Kotz, Peptides that regulate food
tegmental cholinergic neurons that activate dopamine neurons, Behav.
intake: regional, metabolic, and circadian specificity of lateral hypothalamic
Neurosci. 107 (1993) 1077–1087.
orexin A feeding stimulation, Am. J. Physiol. Regul. Integr. Comp. Physiol. 284
[366] M.R. Yeomans, R.W. Gray, Selective effects of naltrexone on food pleasantness
and intake, Physiol. Behav. 60 (1996) 439–446.
[339] M.G. Tordoff, Obesity by choice: the powerful influence of nutrient
[367] M.R. Yeomans, P. Wright, Lower pleasantness of palatable foods in
availability on nutrient intake, Am. J. Physiol. Regul. Integr. Comp. Physiol.
nalmefene-treated human volunteers, Appetite 16 (1991) 249–259.
282 (2002) R1536–R1539.
[368] D.S. Zahm, Functional–anatomical implications of the nucleus accumbens
[340] K. Touzani, R. Bodnar, A. Sclafani, Activation of dopamine D1-like receptors in
core and shell subterritories, Ann. N.Y. Acad. Sci. 877 (1999) 113–128.
nucleus accumbens is critical for the acquisition, but not the expression, of
[369] D.S. Zahm, An integrative neuroanatomical perspective on some subcortical
nutrient-conditioned flavor preferences in rats, Eur. J. Neurosci. 27 (2008)
substrates of adaptive responding with emphasis on the nucleus accumbens,
Neurosci. Biobehav. Rev. 24 (2000) 85–105.
[341] A.L. Tracy, D.J. Clegg, J.D. Johnson, T.L. Davidson, S.C. Benoit, The melanocortin
[370] T. Zetterstrom, T. Sharp, A.K. Collin, U. Ungerstedt, In vivo measurement of
antagonist AgRP (83–132) increases appetitive responding for a fat, but not a
extracellular dopamine and DOPAC in rat striatum after various dopamine-
carbohydrate, reinforcer, Pharmacol. Biochem. Behav. 89 (2008) 263–271.
releasing drugs; implications for the origin of extracellular DOPAC, Eur. J.
[342] D. Treit, K.C. Berridge, A comparison of benzodiazepine, serotonin, and
Pharmacol. 148 (1988) 327–334.
dopamine agents in the taste-reactivity paradigm, Pharmacol. Biochem.
[371] M. Zhang, C. Balmadrid, A.E. Kelley, Nucleus accumbens opioid, GABaergic,
Behav. 37 (1990) 451–456.
and dopaminergic modulation of palatable food motivation: contrasting
[343] M. Tschop, D.L. Smiley, M.L. Heiman, Ghrelin induces adiposity in rodents,
effects revealed by a progressive ratio study in the rat, Behav. Neurosci. 117
Nature 407 (2000) 908–913.
(2003) 202–211.
[344] T.M. Tzschentke, Measuring reward with the conditioned place preference
[372] M. Zhang, B.A. Gosnell, A.E. Kelley, Intake of high-fat food is selectively
(CPP) paradigm: update of the last decade, Addict. Biol. 12 (2007) 227–462.
enhanced by mu opioid receptor stimulation within the nucleus accumbens,
[345] U. Ungerstedt, Is interruption of the nigrostriatal dopamine system producing
J. Pharmacol. Exp. Ther. 285 (1998) 908–914.
the ‘‘lateral hypothalamic syndrome"?, Acta Physiol. Scand. 80 (1970) 35A–
[373] M. Zhang, A.E. Kelley, Opiate agonists microinjected into the nucleus
accumbens enhance sucrose drinking in rats, Psychopharmacology (Berl.)
132 (1997) 350–360.
psychostimulant reward and related behaviors, Neurosci. Biobehav. Rev. 18
[374] M. Zhang, A.E. Kelley, Enhanced intake of high-fat food following striatal mu-
(1994) 207–214.
[347] J.M. van Ree, D. de Wied, Involvement of neurohypophyseal peptides in drug-
Neuroscience 99 (2000) 267–277.
mediated adaptive responses, Pharmacol. Biochem. Behav. 13 (Suppl. 1)
[375] H. Zheng, L.M. Patterson, H.R. Berthoud, Orexin signaling in the ventral
(1980) 257–263.
tegmental area is required for high-fat appetite induced by opioid
[348] C. Vaughan, M. Moore, C. Haskell-Luevano, N.E. Rowland, Food motivated
stimulation of the nucleus accumbens, J. Neurosci. 27 (2007) 11075–11082.
behavior of melanocortin-4 receptor knockout mice under a progressive ratio
[376] F.M. Zhou, C.J. Wilson, J.A. Dani, Cholinergic interneuron characteristics and
schedule, Peptides 27 (2006) 2829–2835.
nicotinic properties in the striatum, J. Neurobiol. 53 (2002) 590–605.
[349] J.G. Veening, S. Te Lie, P. Posthuma, L.M. Geeraedts, R. Nieuwenhuys, A
[377] J.M. Zigman, J.E. Jones, C.E. Lee, C.B. Saper, J.K. Elmquist, Expression of ghrelin
topographical analysis of the origin of some efferent projections from the
receptor mRNA in the rat and the mouse brain, J. Comp. Neurol. 494 (2006)
lateral hypothalamic area in the rat, Neuroscience 22 (1987) 537–551.
Source: http://enosi.ca/data/documents/Fulton.pdf
Know Your FSA /HSA Eligible and Ineligible Expenses Maximize the Value of Your Reimbursement Account Your Flexible Spending Account (FSA) and Health Savings Account (HSA) dol ars can be used for a variety of out-of-pocket health care expenses. Take a look at the fol owing lists for a better understanding of what is and is not eligible. Eligible Expenses
Fall 2012 Edition Website Updates Personalizing Tamoxifen Therapy in London The Lawson Translational Cancer Research Team (LTCRT) based in London has been facilitating the development and adoption of personalized cancer medicine using pharmacogenomics. Dr. Richard Kim, a recipient of the Cancer Care Ontario (CCO) Research Chair (Tier-1) Award in 2010 and leader of a program of Personalized Medicine at the London Health Sciences Centre, has been working closely with a team of oncologists and the LTCRT to ensure a group of breast cancer patients experience the best outcomes possible. Tamoxifen is an important drug for the treatment and prevention of certain breast cancers, and is metabolized in the liver by a drug metabolizing enzyme called CYP2D6. This enzyme is responsible for converting tamoxifen to its active form, endoxifen. The CYP2D6 enyzme occurs in different forms in human beings (called "polymorphisms") which may metabolize the same drugs differently. There is compelling research that shows that women with less active variants of CYP2D6 are at greater risk for breast cancer recurrence compared to those without such polymorphisms when treated with tamoxifen. In March of 2010, Dr. Kim started a personalized medicine clinic for breast cancer patients on tamoxifen therapy using the research funds available through his CCO Chair award. Patients referred to his clinic have their CYP2D6 genotyped as well as tamoxifen and endoxifen blood levels assessed using state-of-the-art genotyping and drug level analysis technologies. Dr. Kim then provides a detailed report to the referring oncologist in terms of the patient's ability to metabolize tamoxifen. He now has data from over 200 patients that show not only are CYP2D6 polymorphisms important to tamoxifen bioactivation to endoxifen, but so are polymorphisms in a another enzyme (CYP3A4) and the patient's vitamin D level.