Toxicity research report final-
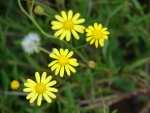
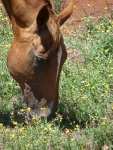
Fireweed Toxicity
Facts and
Steven M Colegate BSc(Hons), PhD
Photos: Forest&Kim Starr
Fireweed Toxicity
Facts and Perspectives
Executive Summary
• HPLC-MS analysis of fireweed collected in the Bega Valley (NSW) in the
spring of 2006 and 2008 showed the presence of dehydropyrrolizidine alkaloid
esters at levels up to about 220 milligrams per kilogram of plant.
• Due to the adverse effects of dehydropyrrolizidine alkaloid esters, a
comprehensive assessment of livestock health, welfare and productivity in the
infested areas should be undertaken.
• Animal-derived food for humans, especially bee-products and honey produced
by bees foraging on fireweed, require monitoring for the presence of fireweed
pyrrolizidine alkaloids.
• All possible measures should be undertaken to manage and control the
infestation and potential spread of fireweed in Australia.
Fireweed Toxicity
Facts and Perspectives
• Senecio madagascariensis (fireweed) is a non-indigenous invasive weed that
is expanding its range in Australia.
• It is closely related to the indigenous Senecio lautus in both morphology and
its distribution.
• The dehydropyrrolizidine alkaloid esters have been associated with acute and
chronic liver toxicity, pneumotoxicity, carcinogenicity and genotoxicity.
• Toxic dehydropyrrolizidine alkaloids have been detected in samples of
fireweed collected from the Bega Valley in the spring of 2006 and 2008.
• The alkaloid profiles of young plant and mature green-stemmed plant and
mature red-stemmed plant are very similar.
• In this limited survey, young plants contain higher levels (up to about 220
milligrams per kilogram of plant) of the toxic pyrrolizidine alkaloids than the
mature plant (up to about 110 milligrams per kilogram of plant).
• The presence of alkaloids was confirmed using ion trap mass spectrometry and
time of flight mass spectrometry. Additionally a sample was analyzed by
another laboratory experienced in Senecio madagascariensis.
• The suspected presence of a low level of a toxic pyrrolizidine alkaloid,
associated with fireweed, in a single, blended wild honey sample of unclear
heritage remains unconfirmed.
Fireweed Toxicity
Facts and Perspectives
CONTENTS
Executive Summary . 1 Executive Summary . 2 Summary . 3 List of Figures . 5 Introduction . 7 Potential Toxicity Issues . 8
Toxicology of Dehydropyrrolizidine Alkaloids (DHPAs) . 8
Potential Toxicity of DHPAs for Livestock . 11 Potential Toxicity of DHPAs for Humans . 12
Relevance of DHPA Content and Toxicity to Fireweed . 13 General Approach and Examples . 16 Analysis of Fireweed . 19
Ion trap mass spectrometry . 19 Time-of-flight mass spectrometry . 24
Analysis of Wild Honey from the Bega Valley . 27
Conclusions and Recommendations . 28 References . 30
Fireweed Toxicity
Facts and Perspectives
List of Figures
Figure 1:
Common structural cores of dehydropyrrolizidine alkaloid esters
Figure 2:
Structures of pyrrolizidine alkaloids isolated from Senecio
madagascariensis sourced from Hawaii and Australia (Gardner et al.,
2006). The bold number is the mass of the molecular ion adduct
(MH+) for each alkaloid.
Figure 3:
HPLCesiMS ion chromatograms showing alkaloidal profiles for
Echium plantagineum flowers (A) and leaves (B). Each of the peaks
represents a different pyrrolizidine alkaloid. In this case the alkaloids
are in their N-oxide form.
Figure 4:
Base ion (m/z 200 – 500) HPLC-ESI-MS chromatograms for (A)
pollen loads from bees foraging on Echium vulgare; (B) pollen loads
from bees foraging on Eupatorium cannabinum; (C) pollen, hexane-
washed from anthers of Senecio jacobaea; and (D) pollen loads from
bees foraging on Senecio ovatus. The internal standard (IS) is used to
standardize the chromatograms. The presence of different suites of
alkaloids from each sample is evident. The alkaloids are in their N-
Figure 5:
The HPLC-MS ion chromatogram of an extract of honey produced by
bees that foraged in the vicinity of Echium vulgare. Each of the six
"arrowed" peaks is a hepatotoxic pyrrolizidine alkaloid that is also
found in the plant itself.
Figure 6:
HPLC-esiMS base ion (m/z 200-500) chromatograms of the alkaloidal
components of Senecio madagascariensis (fireweed): A. fresh young
plant; B. fresh mature plant, and C. dried and milled mature plant.
Figure 7:
HPLC-esi-ion trap MS base ion (m/z 200-500) chromatograms of: A.
fresh young fireweed; B. fresh mature fireweed; and C. dried and
milled mature fireweed. Also shown are example mass spectra for
selected peaks: a. Unidentified alkaloid, m/z 424 (1); b. N-oxide of
senecionine type alkaloid, m/z 352 (2); c. N-oxide of a retrorsine type
alkaloid, m/z 368 (3).
Fireweed Toxicity
Facts and Perspectives
Figure 8:
HPLC-esi-ion trap MS base ion chromatograms of: A. an extract of
young Senecio madagascariensis spiked with; B. integerrimine-N-
oxide, C. usaramine, D. retrorsine, and E. senecionine-N-oxide.
Figure 9:
An HPLC-MS comparison of a zinc/acid-reduced extract of fireweed
collected in the Bega Valley, New South Wales in about October 2006
with that collected in northern New South Wales (Gardner et al.,
2006). The bold numbers are the MH+ values for each peak (see
Figure 2). The analyses were done by Dr Dale Gardner of the USDA
Poisonous Plants Research Laboratory in Logan, Utah, USA
Figure 10:
Direct infusion, ESI-TOF MS analysis of fresh Senecio
madagascariensis collected in the Bega Valley, October 2008. A.
young plants; B. mature, red-stemmed plants; and C. mature, green-
Figure 11.
HPLC-TOF mass spectrometric analysis of fresh, green-stemmed
mature samples (green trace) and fresh, red-stemmed mature samples
(red trace) of Senecio madagascariensis. The numbers shown are the
masses of the ions observed.
Fireweed Toxicity
Facts and Perspectives
Introduction
In North America, "fireweed" is a trivial name used for the purple-flowered
Epilobium angustifolium and Erechtites hieracifolia. In Australia, in addition to
"fireweed" being used to describe a toxic blue-green algae (Lyngbya majuscule),
"fireweed" can be a generic, trivial name applied to a number of weed species in the
Senecio genus of the Asteraceae (daisy/thistle) Family. In the context of this report
"fireweed" refers to Senecio madagascariensis Poiret that is spreading prolifically
along the east coast of Australia and specifically, in the particular context of this
report, the Bega Valley in New South Wales where it is a declared plant under the
Noxious Weeds Act 1993 (www.dpi.nsw.gov.au/agriculture/ pests-
Senecio madagascariensis, a native of South Africa, is closely related to S. lautus
which is native to Australia
(www.esc.nsw.gov.au/weeds/Sheets/herbs/H%20Fireweed.htm) and is one of over
1200 Senecio species world-wide. It was first observed in the Hunter Valley region of
Australia in about 1918. Apparently, it is not a declared noxious plant in this region
Since Senecio spp. produce alkaloids that are toxic to livestock and humans, and since
previous phytochemical analyses of S. madagascariensis in particular have shown the
presence of hepatotoxic (liver damaging) alkaloids, it was deemed essential to
complete a phytochemical assessment of the fireweed collected in the Bega Valley of
New South Wales.
This report, and the research described within, have been completed at the request of
the New South Wales, Bega Valley Fireweed Committee and is intended to be
complementary to related reports (also commissioned by the Bega Valley Fireweed
Committee) that describe other aspects of this plant such as its identification, growth
habits, dispersal rates and distribution, and methods of control (if not eradication)
including biological options for control.
Fireweed Toxicity
Facts and Perspectives
Herein, potential problems associated with the expected presence of
dehydropyrrolizidine alkaloid (DHPA) esters in the fireweed are discussed. The
results of a limited phytochemical research project aimed at identifying the
complement of toxic DHPAs in the fireweed and in wild honey gathered in the
vicinity of fireweed are presented and discussed.
Potential Toxicity Issues
The potential toxicity issues for livestock and humans associated with fireweed are
due to the likely presence of DHPA esters. This diverse class of natural chemicals
comprises over 400 different entities occurring in over 6000 species of plants world-
The basic structural variations of the alkaloids include monoesters, open chain
diesters and macrocyclic diesters of the free base and corresponding N-oxide forms of
dehydropyrrolizidine-based and the otonecine-based alkaloids (Figure 1).
There exists a high level of variation in the suite of DHPAs found in different plant
genera and different species. There can even be variation in the DHPA complement
between different populations of the same species.
Toxicology of Dehydropyrrolizidine Alkaloids (DHPAs)
Following absorption into the blood stream, the DHPA esters are rapidly metabolized
by the liver to form the didehydropyrrolizidine alkaloid form commonly referred to as
the "pyrrolic" form. It is this pyrrolic form that then reacts with macromolecules
within the liver (eg., proteins and DNA) to initiate the chain of events culminating in
clinical disease (Stegelmeier et al., 1999).
The DHPAs are primarily liver toxins (hepatotoxic) but can also "spill out" of the
liver to damage the lungs (pnuemotoxic). In some animals, some DHPAs have been
shown to cause cancers (carcinogenic) and damage genes (mutagenic).
Fireweed Toxicity
Facts and Perspectives
Not all DHPAs are as toxic as each other. Not all animal species are equally
susceptible to the DHPAs. This is due, in the main, to the relative ease (or difficulty)
with which the DHPAs are metabolized to the reactive pyrrolic forms. For example,
sheep are relatively resistant to the effects of DHPAs and, as such, have been
recommended as grazing control options (Schmidl, 2006; www.dpi.nsw.gov.au
/ data/assets/pdf_file/0007/49840 /fireweed _-_primefact_126-final.pdf). On the
other hand, cattle and horses are relatively susceptible to the toxic effects of ingested
Even without knowing the reasons why, the toxicity of Senecio spp. to livestock was
first recognized in the early 20th century. Similarly, the danger to humans was first
recognized in 1920 when cirrhosis of the liver was attributed to senecio poisoning
(Willmot and Robertson, 1920).
Subsequently there has been extensive research into the detection of DHPAs and the
determination of their mechanism of action and their toxicities. A relatively recent
review states: "Pyrrolizidine alkaloids are the leading plant toxins associated with
disease in humans and animals. The PAs present a serious risk to human populations
. Some PA adducts are persistent in animal tissues and the metabolites may be
released and cause damage long after the initial period of ingestion. " (Prakash et
al.,1999).
Fireweed Toxicity
Facts and Perspectives
Macrocyclic diester
Otonecine diester
Figure 1: Common structural cores of dehydropyrrolizidine alkaloid esters
Fireweed Toxicity
Facts and Perspectives
Potential Toxicity of DHPAs for Livestock
The acute and chronic effects of exposure of livestock to dietary DHPAs have been
well-documented (for example: Stegelmeier et al., 1999). The major effect is on the
liver and many clinical signs are related to this initial insult. For example, chronic
poisoning of horses by dietary DHPAs causes a neurological syndrome that is related
to increased levels of systemic ammonia (particularly in the brain) as a result of the
damaged liver's decreased capacity to process ammonia to urea.
While cattle, horses, pigs and chickens are quite susceptible to the poisonous effects
of the DHPAs, sheep and goats are fairly resistant. However, sheep are very
susceptible to copper poisoning and exposure to DHPAs can exacerbate the
accumulation of copper in the liver of the sheep. Eventually an acute, copper-induced
haemolytic crisis occurs, as the red blood cells are disrupted, causing the death of the
animal. Clinical signs of chronic exposure to DHPAs vary depending upon the animal
exposed but can be attributed to the loss of liver function. Signs of exposure can
include a rough, unkempt appearance, oedema of gastro-intestinal tract, ascites (fluid-
filled body cavities eg the peritoneal cavity), diarrhea, prolapsed rectum, dullness and
lethargy, photosensitization and general abnormal behaviour. As the liver disease
progresses then jaundice will be observed. A summary of clinical signs resulting from
exposure to DHPAs is presented in the Merck Veterinary Manual
On post-mortem examination, the livers in most species are shrunken, hard to the
touch and fibrotic in nature following chronic exposure to the DHPAs. In sheep,
however, the livers can be soft and mushy rather than hard. Microscopic indicators of
exposure to the DHPAs include the observation of enlarged liver cells (hepatocytes)
referred to as megalocytosis.
The long term prognosis for affected livestock is not good due to the primary effect on
the liver. It has also been shown that the fatal effects can be delayed with no
immediate signs of liver damage following the initial exposure. For example, steers
given a single sub-lethal dose of Senecio riddellii, S. jacobaea or S. longilobus died
from a progressive liver failure several months later (Molyneux et al., 1988).
Fireweed Toxicity
Facts and Perspectives
Using sheep as grazing control agents for the DHPA-producing plants is not without
its risks to the sheep since, although relatively resistant to the effects of the DHPAs,
they are not totally resistant. As a result, the use of sheep as biocontrol agents may be
unacceptable on animal welfare grounds (www.bbc.co.uk/dna/h2g2/A22548774). It
has not been positively confirmed that such "biocontrol" animals do not accumulate
toxic metabolites of the DHPAs in meat destined for the human food supply.
The lethal effects (and economic losses associated with them) of acute or chronic
exposure of livestock to the DHPAs are not expected to be the major source of loss to
producers. Low-level, sub-clinical exposures can lead to severe losses in productivity
that may include poor feed utilization and reduced weight gains, reduced milk yields
and potential reproductive/breeding adverse effects.
Potential Toxicity of DHPAs for Humans
A review published in 1989 clearly demonstrated the exposure of humans to the
DHPAs and the adverse consequences (Huxtable, 1989). Since then, there have been
continuing reports of humans being poisoned by dietary DHPAs, including exposure
via medicinal herbs preparations, cooking spices and flour made from grain
contaminated with seeds from DHPA-producing plants.
The major, reported clinical symptoms of pyrrolizidine alkaloid intoxication in
humans result from a veno-occlusive disease of the liver (Prakash et al., 1999). In
addition to outbreaks of "bread poisoning", when the seeds of pyrrolizidine alkaloid-
producing plants were co-harvested with milling grains (Ahmad, 2001), pyrrolizidine
alkaloids in herbs and spices have been associated with many instances of fatal
hepatic disease in adult, neonatal and prenatal humans. Whilst the effects of acute
exposure to pyrrolizidine alkaloids on humans and animals have been well
documented, the effects of long term, low level exposure to DHPAs via the diet
(grain, milk, meat, honey, and related products) (Colegate et al., 1998) are a relatively
unknown factor. In addition, and for many reasons, adverse clinical effects can be
very difficult to unambiguously associate with the sources of dietary DHPAs.
After a comprehensive risk assessment of toxic pyrrolizidine alkaloids, the German
Federal Health Bureau established regulations that restrict oral exposure to
Fireweed Toxicity
Facts and Perspectives
pyrrolizidine alkaloids or their N-oxides in herbal preparations to 0.1 microgram per
day with the exclusion of pregnant and lactating women for which zero exposure is
recommended (German Federal Health Bureau, 1992). In the Netherlands, levels of
pyrrolizidine alkaloids and their N-oxides are restricted to 0.1 microgram per 100 g of
food (H van Egmond, The Netherlands National Institute for Public Health and the
Environment, personal communication). In Australia, the Food Standards Australia
New Zealand (FSANZ, 2001) has set a limit of exposure to 1 microgram per kilogram
bodyweight per day. For example, a 30 kg child would be allowed 30 micrograms of
DHPAs (or their N-oxides) per day. In contrast to the European deliberations, the
FSANZ did not consider DHPA-induced carcinogenicity or genotoxicity as a major
factor with humans and therefore developed their recommendations on the occurrence
(or prevention of the occurrence) of hepatic veno-occlusive disease.
In addition to the clearly proven contamination of grains, and the use of cooking
spices and medicinal herbs either prepared or contaminated with DHPA-producing
plants, the potential exists for humans to be exposed to DHPAs (and their N-oxides)
via honey and bee products (Edgar et al., 2001; Beales et al., 2004; Betteridge et al.,
2005; Boppré et al., 2005), milk (James et al., 1994), eggs (Edgar and Smith, 2000)
and meat (Seawright, 1994).
Relevance of DHPA Content and Toxicity to Fireweed
In Australia, grazing of fireweed (Senecio madagascariensis)-contaminated pasture
has previously been associated, but not proven, with livestock poisonings in New
South Wales (Seaman and Walker, 1985). Another report describes pyrrolizidine
alkaloidosis in a 2 month-old foal and associated it with in utero exposure of the
foetus to DHPAs ingested by the mare grazing a pasture heavily infested with
fireweed (Small et al., 1993). An intoxication of cattle was associated with S. lautus
(Noble et al., 1994) but, apparently, subsequent re-examination of the herbarium
specimens identified the plant as S. madagascariensis (referred to in Gardner et al.,
Despite the previous associations of fireweed with overt disease of livestock, it was
only recently that the DHPAs, in fireweed collected in Hawaii and in Australia, were
Fireweed Toxicity
Facts and Perspectives
identified and compared (Gardner et al., 2006). This report, that used gas
chromatography-mass spectrometry (GCMS) to identify 12 macrocyclic DHPAs
(Figure 2) at levels that were potentially toxic to livestock (217 – 1990 micrograms
per gram of plant material). The DHPA profile for the fireweed collected in Hawaii
was essentially identical to the profile determined for a composite sample of fireweed
collected from northern New South Wales. Citing this latter observation the authors
supported the contention that fireweed in Hawaii arrived via Australia.
The research by Gardner et al (2006) on the fireweed in Hawaii highlighted the high
variation in DHPA content and profile from plants collected at different locations.
Therefore, to help complete the understanding of the fireweed population, and
problems posed by it, in the Bega Valley area of New South Wales, a limited
phytochemical analysis of the plants collected in the area was conducted.
Fireweed Toxicity
Facts and Perspectives
Desacetyldoronine
Acetylsenkirkine
Figure 2: Structures of pyrrolizidine alkaloids isolated from Senecio
madagascariensis sourced from Hawaii and Australia (Gardner et al., 2006). The
bold number is the mass of the molecular ion adduct (MH+) for each alkaloid.
Fireweed Toxicity
Facts and Perspectives
Analysis for Dehydropyrrolizidine Alkaloids
In mid to late spring of 2006, samples of mature, flowering plants and juvenile plants
were collected in the Bega Valley and immediately sent to Dr Steven Colegate,
Leader of the CSIRO Plant-associated Toxins Research Laboratory in Geelong,
Victoria, for processing and analysis. In mid spring of 2008 other samples of plants
and samples of wild honey collected in the same area were sent to Dr Steven Colegate
for analysis at Deakin University once the CSIRO had closed down its plant toxins
research group. The plants included mature flowering plants, some with a red hue to
the stems and some just green, and juvenile plants. There is no botanical separation at
this stage of the green-stemmed fireweed and the red-stemmed fireweed.
All samples were received in a fresh condition and were immediately processed for
The earlier samples processed at CSIRO were analysed using high pressure liquid
chromatography (HPLC)-electrospray ionization (ESI) ion trap mass spectrometry
(MS) while the samples analyzed at Deakin University were processed using HPLC-
ESI-time of flight (TOF) MS.
General Approach and Examples
The plant and honey samples were analyzed for the presence of DHPAs using a
sequential combination of solvent extraction, solid phase concentration and
subsequent high pressure liquid chromatography-mass spectrometry (HPLC-MS) in a
manner previously described for:
• plants eg., Echium plantagineum (Paterson's Curse, Salvation Jane) (Colegate
et al., 2005) (Figure 3), Echium vulgare, Senecio jacobaea (ragwort) and
Senecio ovatus (Boppré et al., 2008)
• pollen collected from plants or bees eg., Echium vulgare (Boppré et al.,
2005), Eupatorium cannabinum, Senecio jacobaea and Senecio ovatus
(Boppré et al., 2008) (Figure 4)
• honey (Betteridge et al., 2005) (Figure 5)
Fireweed Toxicity
Facts and Perspectives
In all examples shown, and in the analyses of fireweed in the following section, the
individual DHPAs are represented by peaks in an ion chromatogram. Identities are
rationalized using the mass spectrometric data collected for each peak and subsequent
comparison with authentic standards of those alkaloids or with mass data reported in
the scientific literature. In general, because the alkaloids within a species of plant are
structurally similar, the size of the peak reflects the relative abundances of the
individual pyrrolizidine alkaloids in the sample.
Figure 3:
HPLCesiMS ion chromatograms showing alkaloidal profiles for
Echium plantagineum flowers (A) and leaves (B). Each of the peaks represents a
different pyrrolizidine alkaloid. In this case the alkaloids are in their N-oxide form.
In contrast to the GC-MS method employed by Gardner et al (2006), this HPLC-MS
method of analysis allows the simultaneous extraction, recovery and analysis of the
pyrrolizidine alkaloids and their N-oxides. Since the N-oxides are also toxic (Chou et
al., 2003) it is important that they are fully accounted.
The ion chromatograms shown in Figure 3 demonstrate how different parts of the
same plant can have slightly different alkaloid profiles. In this case the flowers
produce acetylated derivatives of the alkaloid-N-oxides detected in the leaves
(Colegate et al., 2005). However, Figure 4 clearly shows the different profiles of
Fireweed Toxicity
Facts and Perspectives
Time
(min)
Figure 4:
Base ion (m/z 200 – 500) HPLC-ESI-MS chromatograms for (A)
pollen loads from bees foraging on Echium vulgare; (B) pollen loads from bees
foraging on Eupatorium cannabinum; (C) pollen, hexane-washed from anthers of
Senecio jacobaea; and (D) pollen loads from bees foraging on Senecio ovatus. The
internal standard (IS) is used to standardize the chromatograms. The presence of
different suites of alkaloids from each sample is evident. The alkaloids are in their N-
oxide forms.
alkaloids that might be expected from different plant genera i.e., Echium versus
Senecio versus Eupatorium, and even from different species of the same genus i.e.,
Senecio jacobaea versus Senecio ovatus (Boppré et al., 2008).
As a final example of the application of this methodology, Figure 5 shows the
detection of hepatotoxic pyrrolizidine alkaloids detected in honey produced by bees
that foraged in the vicinity of the DHPA-producing plant Echium vulgare (Betteridge
et al., 2005). The levels detected in this commercial honey would clearly exceed
European and Australian regulations.
Fireweed Toxicity
Facts and Perspectives
22/05/2004 12:04:15
Honey N ov.2002-5(Arataki clover blend) elute 1 (1Eho2)
R T: 0.00 - 19.99
m /z= 200.0-500.0 MS
Figure 5:
The HPLC-MS ion chromatogram of an extract of honey produced by
bees that foraged in the vicinity of Echium vulgare. Each of the six "arrowed" peaks
is a hepatotoxic pyrrolizidine alkaloid that is also found in the plant itself.
Analysis of Fireweed
Samples of fireweed were processed and analysed using two different mass
spectrometry approaches: 1. ion trap mass spectrometry, and 2. time of flight (TOF)
mass spectrometry.
Ion trap mass spectrometry
Qualitatively, there were no major differences observed in the alkaloid profiles of the
mature plant and the juvenile plants collected in 2006 and analyzed using HPLC-ESI-
ion trap MS i.e., the chromatograms looked very similar.
Within the profiles, several, potentially toxic DHPAs were identified (Figure 6) in
extracts of the Bega Valley fireweed. The structures of the alkaloids were indicated by
comparison of their mass spectrum characteristics (for example, Figure 7) with the
Fireweed Toxicity
Facts and Perspectives
500.0 MS yu260906lc
500.0 MS yu260906lc
Figure 6:
HPLC-esi-ion trap MS base ion (m/z 200-500) chromatograms of the
alkaloidal components of Senecio madagascariensis (fireweed): A. fresh young plant;
B. fresh mature plant, and C. dried and milled mature plant.
literature report (Gardner et al., 2006) and using authentic standards (Figure 8) from
the collection of the CSIRO Plant Toxins Research Group.
The major peaks with MH+ ions at m/z 368 and 352 all showed a significant dimer ion
signal at m/z 735 and 703 respectively. This is good evidence for the N-oxide
character (Figure 1) of the alkaloids (Colegate et al., 2005), in this case the N-oxides
of the usaramine-type alkaloids (MH+ 352) and senecionine-type alkaloids (MH+ 336)
respectively (see Figure 2). On the contrary, the major peaks with MH+ ions at m/z
382 and 424 did not show the presence of dimeric ions and this is supportive of their
structures being of the otonecine type (Figure 1) such as otosenine and florosenine
respectively (see Figure 2).
Fireweed Toxicity
Facts and Perspectives
Figure 7:
HPLC-esi-ion trap MS base ion (m/z 200-500) chromatograms of: A.
fresh young fireweed; B. fresh mature fireweed; and C. dried and milled mature
fireweed. Also shown are example mass spectra for selected peaks: a. Unidentified
alkaloid, m/z 424 (1); b. N-oxide of senecionine type alkaloid, m/z 352 (2); c. N-oxide
of a retrorsine type alkaloid, m/z 368 (3).
Fireweed Toxicity
Facts and Perspectives
460.4 461.3 461.4
Base Peak m/z= 200.0-500.0
Figure 8:
HPLC-esi-ion trap MS base ion chromatograms of: A. an extract of
young Senecio madagascariensis spiked with; B. integerrimine-N-oxide, C.
usaramine, D. retrorsine, and E. senecionine-N-oxide.
The alkaloid profile observed for the Bega Valley fireweed samples was similar but
seemed sufficiently different from the report on the profiles of both the Hawaiian and
Australian (northern NSW) samples previously analyzed (Gardner et al., 2006). It was
the apparent close similarity in these latter samples that prompted the conclusion that
the Hawaiian fireweed was originally from Australia. Therefore, a sample of the
extract of the Bega Valley plants was sent to Dr Dale Gardner of the USDA
Poisonous Plants Laboratory in Logan, Utah, USA for comparative analysis with their
sample of Australian fireweed collected in northern New South Wales (Figure 9).
Fireweed Toxicity
Facts and Perspectives
RT: 0.00 - 20.00 SM: 3B
Northern NSW
0.40 1.73 2.43 2.87
17.04 17.28 19.69
Bega Valley
350 10.07
350 8.26 8.80
3.08 4.65 5.44 5.55 6.97
Figure 9: An HPLC-MS comparison of a zinc/acid-reduced extract of fireweed
collected in the Bega Valley, New South Wales in about October 2006 with that
collected in northern New South Wales (Gardner et al., 2006). The bold numbers are
the MH+ values for each peak (see Figure 2). The analyses were done by Dr Dale
Gardner of the USDA Poisonous Plants Research Laboratory in Logan, Utah, USA.
The major differences evident between the two Australian samples include the greater
levels of the senecionine-type alkaloids (MH+ 336, Figure 2), the relative imbalance
of the retrorsine-type alkaloids (MH+ 352, Figure 2) and the apparent appearance of
dehydroretrorsine-type alkaloids (MH+ 350) in the Bega Valley sample. The latter are
possibly related to seneciphylline and have not been previously reported in this plant.
Fireweed Toxicity
Facts and Perspectives
In a similar way to the quantitation of alkaloids in honey and pollen (Betteridge et al.,
2005; Boppré et al., 2005; Boppré et al., 2008), the approximate concentrations of the
DHPAs and their N-oxides were estimated by comparison to calibration curves
generated using the authenticated DHPA lasiocarpine and lasiocarpine-N-oxide
respectively. Thus, fresh young plant was estimated to contain between 100 and 220
ppm (milligrams of alkaloids per kilogram of plant) and mature fresh plant between
70 and 110 ppm. Another sample of mature plant that was dried and milled to a fine
powder was estimated to contain between 140 and 170 ppm of pyrrolizidine alkaloids.
In this very limited survey, it appears that young fresh plant contains more
pyrrolizidine alkaloids than the fresh mature plant. The higher levels estimated in the
dried and milled mature plant reflects the increased efficiency of extraction.
Significantly, there was no apparent selective degradation of most of the alkaloids
during the drying process however, significant loss of the alkaloid with MH+ 424 did
seem to occur. This observation would require further checking for confirmation.
The levels of pyrrolizidine alkaloids detected in the Bega Valley samples was at the
lower end of the scale (217 – 1990 ppm) for the levels detected in samples collected
from various sites in Hawaii (Gardner et al., 2006). It is unlikely that this results from
differences in extraction efficiency but may reflect the relatively limited sampling
procedure employed for the Bega Valley samples, combined with inter-plant
differences in levels.
Time-of-flight mass spectrometry
Samples of fresh fireweed (entire plant) were extracted in the usual way and analyzed
using TOF mass spectrometry to complement the results obtained using ion trap mass
spectrometry. Three plant samples, collected in spring of 2008, were analyzed: 1.
mature fireweed plants with green stems; 2. mature fireweed plants with red stems;
and 3. small (juvenile) fireweed plants.
Fireweed Toxicity
Facts and Perspectives
+ Scan (0.182 min) JFW100.d
Counts vs. Mass-to-Charge (m/z)
+ Scan (0.194 min) RMFW100.d
Counts vs. Mass-to-Charge (m/z)
Figure 10:
Direct infusion, ESI-TOF MS analysis of fresh Senecio
madagascariensis collected in the Bega Valley, October 2008. A. young plants; B.
mature, red-stemmed plants; and C. mature, green-stemmed plants
The direct infusion TOF mass spectrometric analysis (Figure 10) of the three 2008
samples showed the presence of ions compatible with the major pyrrolizidine
alkaloids reported for S. madagascariensis (Gardner et al., 2006) and that were
observed using the ion trap mass spectrometric analysis on plants collected two
seasons earlier in 2006. In particular the ion profiles of the juvenile and mature-red-
stemmed plants were very similar. The ion profile for the mature, green-stemmed
plants was also qualitatively similar to the other samples but it showed a relative
increase in the presence of the ions at 352 and 368. This apparent relative increase
could be a result of the sampling technique i.e., accidentally choosing plants with
slightly different alkaloid profiles, or it could reflect the presence of other alkaloids
with the same molecular weights. The first explanation can be addressed by collecting
more samples and completing seasonal alkaloid profiles of plant populations. The
Fireweed Toxicity
Facts and Perspectives
Figure 11.
HPLC-TOF mass spectrometric analysis of fresh, green-stemmed
mature samples (green trace) and fresh, red-stemmed mature samples (red trace) of
Senecio madagascariensis. The numbers shown are the masses of the ions observed.
second explanation was addressed by examining the high pressure liquid
chromatographic (HPLC) separation of the alkaloids (Figure 11) as with the ion trap
mass spectrometry analysis described for the 2006 samples.
The HPLC-TOF mass spectrometric analysis showed that the alkaloid with a
molecular ion adduct (MH+) of 424 is predominant. In the samples analyzed, the red-
stemmed variation of the plant shows an additional peak with an MH+ of 382 that is
not observed in the green-stemmed variation. The red-stemmed variation also shows
an additional 2 peaks with an MH+ of 368. The green-stemmed variation shows a
larger relative amount of the free alkaloid at 336 (derived from the N-oxide at 352).
Despite these minor differences, the two variations are very similar in the profile of
Fireweed Toxicity
Facts and Perspectives
Analysis of Wild Honey from the Bega Valley
Three small samples of honey-in-the-comb, taken from a wild hive in the vicinity of
previous fireweed flowerings in the Bega Valley, were each extracted with dilute acid.
The three separate extracts were then combined to improve the sensitivity of the
detection method.
The dilute acid extract was processed using solid phase extraction in a slight
modification of the method used to process the plants. The resultant sample was
analyzed using HPLC-TOF-MS.
There was no unambiguous detection of fireweed pyrrolizidine alkaloids in this honey
sample. Nonetheless, the very low level presence in the honey of toxic pyrrolizidine
alkaloids derived from Senecio spp. was indicated by the observation of a peak with
an MH+ of 352 suggestive of senecionine-N-oxide.
The lack of convincing evidence for toxic pyrrolizidine alkaloids in this sample
should not be taken as an indication that fireweed honey generally does not contain
such alkaloids. The actual history of the honey sample presented is unknown and it
may well be that fireweed contributed very little to the foraging bees.
Fireweed Toxicity
Facts and Perspectives
Conclusions and Recommendations
The results of this very limited phytochemical survey of plants collected in the Bega
Valley of New South Wales, clearly demonstrate the presence of hepatotoxic
dehydropyrrolizidine alkaloids. There is increasing international concern about these
alkaloids in animal feed and in the human food supply (European Food safety
Authority, 2007).
On the basis of potential toxicity to livestock and to humans it would seem prudent to
control the spread of fireweed. In the first instance this should involve the rigorous
containment of current populations thereby preventing incursions into new areas.
Where small, invading populations are discovered, they should be immediately
removed. The success of this approach has been reported on Hawaii where, in some
areas, regular monitoring and hand-pulling of fireweed plants has resulted in a
dramatic decrease in the plants observed
(www.hawaiiinvasivespecies.org/iscs/kisc/pdfs/wow3text.pdf). The example set, and
the concern shown, by the Hawaiian Invasive Species Council and the Kauai Invasive
Species Committee (www.hawaiiinvasivespecies.org/iscs/kisc/wow.html) should be
examined and emulated in Australia. Thorne et al (2005) describe an adaptive
management approach to the control of fireweed. The essential component is an
integrated weed management plan comprising three levels of activity i.e., prevention,
control and immediate response. In concert with controlling fireweed where it is
established, the fireweed should be prevented from invading new areas and, when it
does, immediate action should be taken to eradicate it from the new area. Thorne et al
(2005) describe six steps to adaptive management control of fireweed i.e., establish
goals; set management priorities; identify appropriate methods; develop and
implement an integrated weed management plan; monitor results; and adaptive
modification to improve the plan.
Livestock health and productivity should be carefully monitored (taking into
consideration the delayed onset of some clinical signs) in fireweed-endemic areas.
Animal-derived food products, including meat, milk and bee products such as honey
and pollen, should be checked for the presence of hepatotoxic pyrrolizidine alkaloids.
Fireweed Toxicity
Facts and Perspectives
Because of the potential for higher exposures, locally-produced and consumed honey
and pollen products should be specifically monitored for hepatotoxic pyrrolizidine
alkaloid content.
Fireweed Toxicity
Facts and Perspectives
References
Ahmad K (2001). Health and safety of Afghans hangs in the balance. Lancet 358:
Beales KA, Betteridge K, Colegate SM, and Edgar JA (2004). Solid phase extraction
and LCMS analysis of pyrrolizidine alkaloids in honeys. Journal of Agriculture and
Food Chemistry 52: 6664-667.
Betteridge K, Cao Y, and Colegate SM (2005). Improved method of extraction and
LC-MS analysis of pyrrolizidine alkaloids and their N-oxides in honey: Application to
Echium vulgare honeys. Journal of Agriculture and Food Chemistry 53: 1894-1902.
Boppré M, Colegate SM, and Edgar JA (2005). Pyrrolizidine alkaloids of Echium
vulagre honey found in pure pollen. Journal of Agriculture and Food Chemistry 53:
Boppré M, Colegate SM, Edgar JA, Fischer OW (2008) Hepatotoxic pyrrolizidine
alkaloids in pollen and drying-related implications for commercial processing of bee
pollen. J Agric Food Chem 56: 5662-5672.
Chou MW, Wang Y-P, Yan J, Yang Y-C, Beger RD, Williams LD, Doerge DR, and
Fu PP (2003). Riddelliine-N-oxide is a phytochemical and mammalian metabolite
with genotoxic activity that is comparable to the parent pyrrolizidine alkaloid
riddelliine. Toxicol. Lett. 145, 239-247.
Colegate SM, Edgar JA, Knill AM, and Lee ST (2005). Solid phase extraction and
LCMS profiling of pyrrolizidine alkaloids and their N-oxides: A case study of Echium
plantagineum. Phytochemical Analysis 16: 108-119.
Fireweed Toxicity
Facts and Perspectives
Colegate SM, Edgar JA, and Stegelmeier BL (1998). Plant-associated Toxins in the
Human Food Supply. In Environmental Toxicology: Current Developments, Rose J.
(ed.) Gordon and Breach Science Publishers, Amsterdam, pp. 317-344.
Edgar JA and Smith LW (2000). Transfer of pyrrolizidine alkaloids into eggs: Food
safety implications. In Natural and Selected Synthetic Toxins : Biological
Implications, Tu AT, and Gaffield W (eds). American Chemical Society, Washington,
DC, pp. 118-128.
Edgar JA, Roeder E, and Molyneux RJ (2001). Honey from plants containing
pyrrolizidine alkaloids: A potential threat to health. Journal of Agriculture and Food
Chemistry 50: 2719-2730.
European Food Safety Authority. (2007). Opinion of the scientific panel on
contaminants in the food chain on a request from the European Commission related to
pyrrolizidine alkaloids as undesirable substances in animal feed. The EFSA J., 447, 1-
Food Standards Australia New Zealand. 2001. Pyrrolizidine alkaloids in food: a
toxicological review and risk assessment. (http://www.foodstandards.gov.au/_
srcfiles/TR2.pdf ).
Gardner DR, Thorne MS, Molyneux RJ, Pfister JA and Seawright AA (2006).
Pyrrolizidine alkaloids in Senecio madagascariensis from Australia and Hawaii and
assessment of possible livestock poisoning. Biochem. Sys. Ecol. 34: 736-744.
German Federal Department of Health. (1992). Bundesanzeiger June 17: 4805; cited
by: Dtsch Apoth Ztg 132: 1406-1408.
Huxtable RJ (1989). Human health implications of pyrrolizidine alkaloids and herbs
containing them. In Toxicants of Plant Origin, Volume 1 Alkaloids, Cheeke PR (ed).
CRC Press, Boca Raton, Fl., pp. 41-86.
Fireweed Toxicity
Facts and Perspectives
James LF, Panter KE, Molyneux RJ, Stegelmeier BL and Wagstaff DJ (1994). Plant
toxicants in milk. In Plant-associated Toxins: Agricultural, Phytochemical and
Ecological Aspects. Edited by Colegate SM and Dorling PR. CAB International, pp
Molyneux RJ, Johnson AE and Stuart LD. (1988). Delayed manifestation of Senecio-
induced pyrrolizidine alkaloidosis in cattle: case reports. Vet. Hum.Toxicol. 30: 201-
Noble W, Crossley JdeB, Hill BD, Pierce RJ, Mckenzie RA, Debritz M and Morley
AA (1994). Pyrrolizidine alkaloidosis of cattle associated with Senecio lautus. AVJ
Prakash AS, Pereira TN, Reilly PEB, and Seawright AA (1999). Pyrrolizidine
alkaloids in human diet. Mutation Research 443: 53-67.
Seawright, AA (1994). Toxic plant residues in meat. In Plant-associated Toxins:
Agricultural, Phytochemical and Ecological Aspects. Edited by Colegate SM and
Dorling PR. CAB International, pp 77-82.
Seaman JT and Walker KH (1985). Pyrrolizidine alkaloid poisoning of cattle and
horses in New South Wales. In: Plant Toxicology Edited by Seawright AA, Hegarty
MP, James LF and Keeler RF. Queensland Poisonous Plants Committee,
Yeerongpilly. pp. 235-246.
Small AC, Kelly WR, Seawright AA, Mattocks AR and Jukes R (1993). Pyrrolizidine
alkaloidosis in a two month old foal. Zentralbl. Veterinarmed A 40: 213-218.
Stegelmeier BL, Edgar JA, Colegate SM, Gardner DR, Schoch TK, Coulombe RA,
Molyneux RJ. (1999). Pyrrolizidine alkaloid plants, metabolism and toxicity. J. Nat.
Toxins 8, 95 – 116.
Fireweed Toxicity
Facts and Perspectives
Schmidl L (2006). Biology and control of ragwort, Senecio jacobaeaL., in Victoria,
Australia. Weed Res. 12: 37-45.
Thorne MS, Powley JS and Fukumoto GK (2005). Fireweed control: an adaptive
management approach. Pasture and Range Management Oct 2005. Cooperative
Extension Service, College of Tropical Agriculture and Human Resources, University
of Hawaii at Manoa.
Willmot FC and Robertson GW. (1920). Senecio disease, or cirrhosis of the liver due
to senecio poisoning. Lancet 2, 848–849.
Source: http://fireweed.org.au/wp-content/blogs.dir/55/files/2014/04/Toxicity_Research_Report_Final-1_01.pdf
Eur Child Adolesc Psychiatry (2007)16:157–167 DOI 10.1007/s00787-006-0584-x ORIGINAL CONTRIBUTION Childhood depression: a place for Ilan JoffeJesse Campbell Carmen ClementeFredrik Almqvist An outcome study comparing individual psychodynamic psychotherapy and family therapy Ulla Koskenranta-AaltoSheila WeintraubGerasimos KolaitisVlassis TomarasDimitris AnastasopoulosKate GraysonJacqueline BarnesJohn Tsiantis
ProStrakan Announces 3 Abstracts for Sancuso® (Granisetron Transdermal System) Presented at the Multinational Association for Supportive Care in Cancer (MASCC) 2013 Annual Meeting Additional Analyses Further Evaluate Safety and Efficacy of Sancuso in Chemotherapy Induced Nausea and Vomiting (CINV) BRIDGEWATER, NJ—ProStrakan, Inc. ("ProStrakan") announces today (July 9, 2013) that 3 abstracts for Sancuso® (Granisetron Transdermal System) were presented at the Multinational Association for Supportive Care in Cancer (MASCC) 2013 Annual Meeting held June 27-29, in Berlin, Germany. The brand name for granisetron transdermal system (GTS) is SANCUSO (san KOO so). Sancuso is a serotonin subtype 3 (5-HT3) receptor antagonist indicated for the prevention of nausea and vomiting in patients receiving moderately and/or highly emetogenic chemotherapy for up to 5 consecutive days. A patch, Sancuso is applied 24-48 hours prior to chemotherapy, and stays on for up to 7 days, providing 5 full days of complete control of CINV. 1