Bozen2008 169.182
Systems Chemistry, May 26th – 30th, 2008, Bozen, Italy
The Chemistry of Signal Transduction in
Harald Lanig and Timothy Clark
Na¨gelsbachstraße 25, 90152 Erlangen, Germany
Received: 28th October 2008 / Published: 16th March 2009
Signal transduction proteins in biological systems must be very flexibleto undergo the allosteric changes necessary for their function. It iscurrent practice to investigate the modes of action of these systemsby X-ray spectroscopy of the different states trapped as crystals. Un-fortunately, the forces acting on the proteins by packing effects maylead to distortions comparable to the changes that occur during theallosteric movements. This makes it questionable as to whether X-raystructures can be used to deduce induction mechanisms. In this work,we show for DNA-binding tetracycline repressor proteins that molecu-lar dynamics simulations offer an interesting alternative for determin-ing the induction state and possible mechanisms switching betweenthem. Based on data sampled for different repressor classes with sev-eral force field parameter sets, we show that MD simulations haveconvincing advantages over the analysis of static structures influencedby crystal packing.
Signal transduction is the mechanism by which biological processes are turned on and off.
Organisms use signal-transduction pathways to control their development, react to externalstimuli such as heat, cold, excess or lack of nutrients, toxic chemicals etc., but also toregulate cell growth and death. Malfunction of signal-transduction systems can lead, amongothers, to cancer or autoimmune diseases. The effects of many hereditary diseases can also
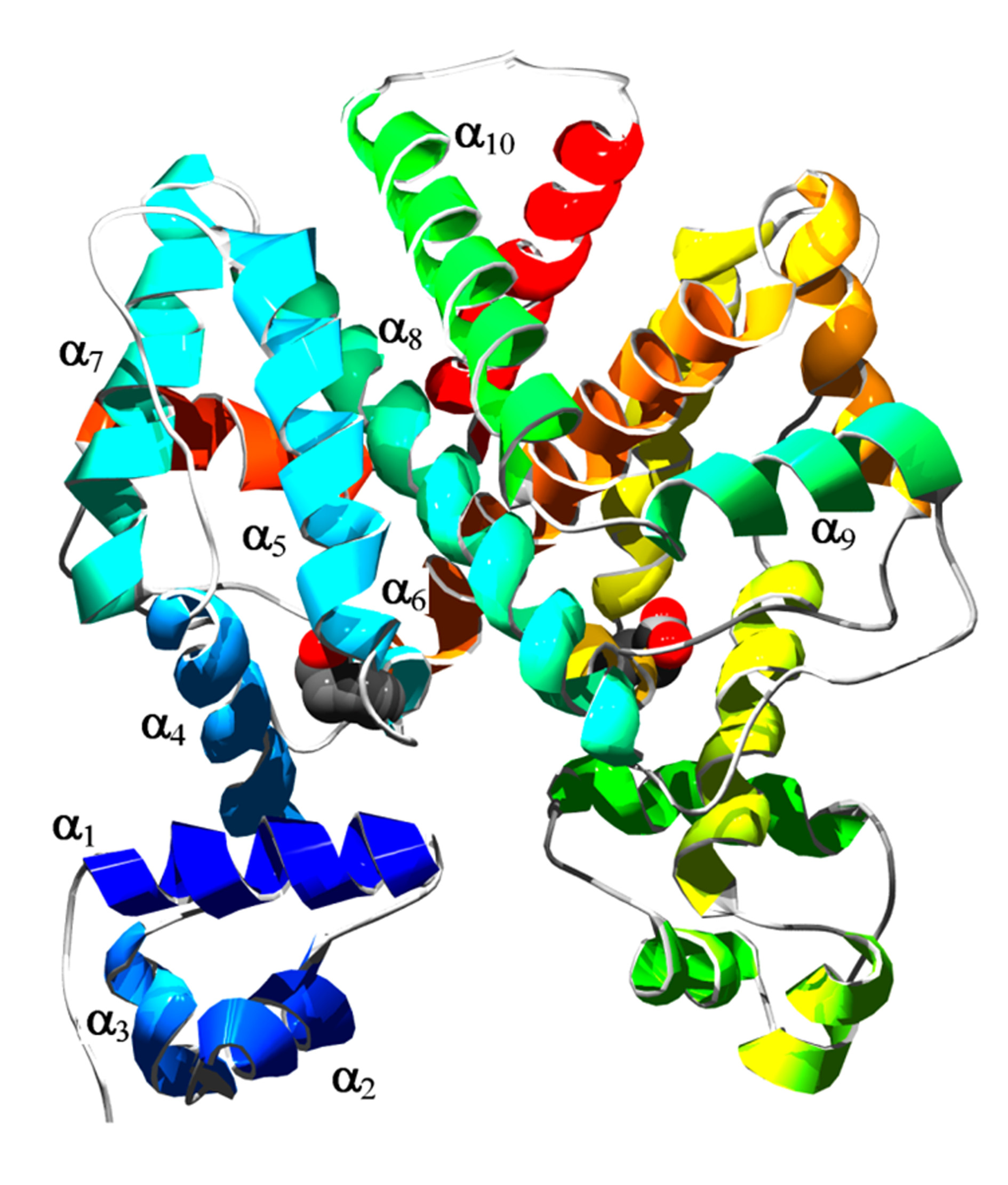
Lanig, H. and Clark, T.
be attributed to malfunction in signal-transduction processes. Thus, signal-transduction path-ways and processes play a major role in the systems chemistry of living systems becausethey combine to form complex signaling networks that control the development, metabolismand defense mechanisms of living organs.
Perhaps the structurally and mechanistically best characterized signal-transduction protein isthe tetracycline repressor (TetR)[1]. TetR switches an efflux-pump defense mechanism [2] inresistant Gram-negative bacteria when they are subjected to tetracycline antibiotics.
The parent compound tetracycline (Tc, shown above) is a natural product produced byseveral Streptomyces strains. The metabolite 5a,6-anhydrotetracycline (ATc, show above)is not an effective antibiotic but induces TetR approximately 500 times better than Tc [3] andis therefore often used in biological experiments rather than a tetracycline antibiotic.
Figure 1. Schematic view of the tetracycline repressor as its homodimer. Thea-helices of the front monomer are numbered. The DNA-binding heads contain ahelix-turn-helix motif and consist of the first three helices.
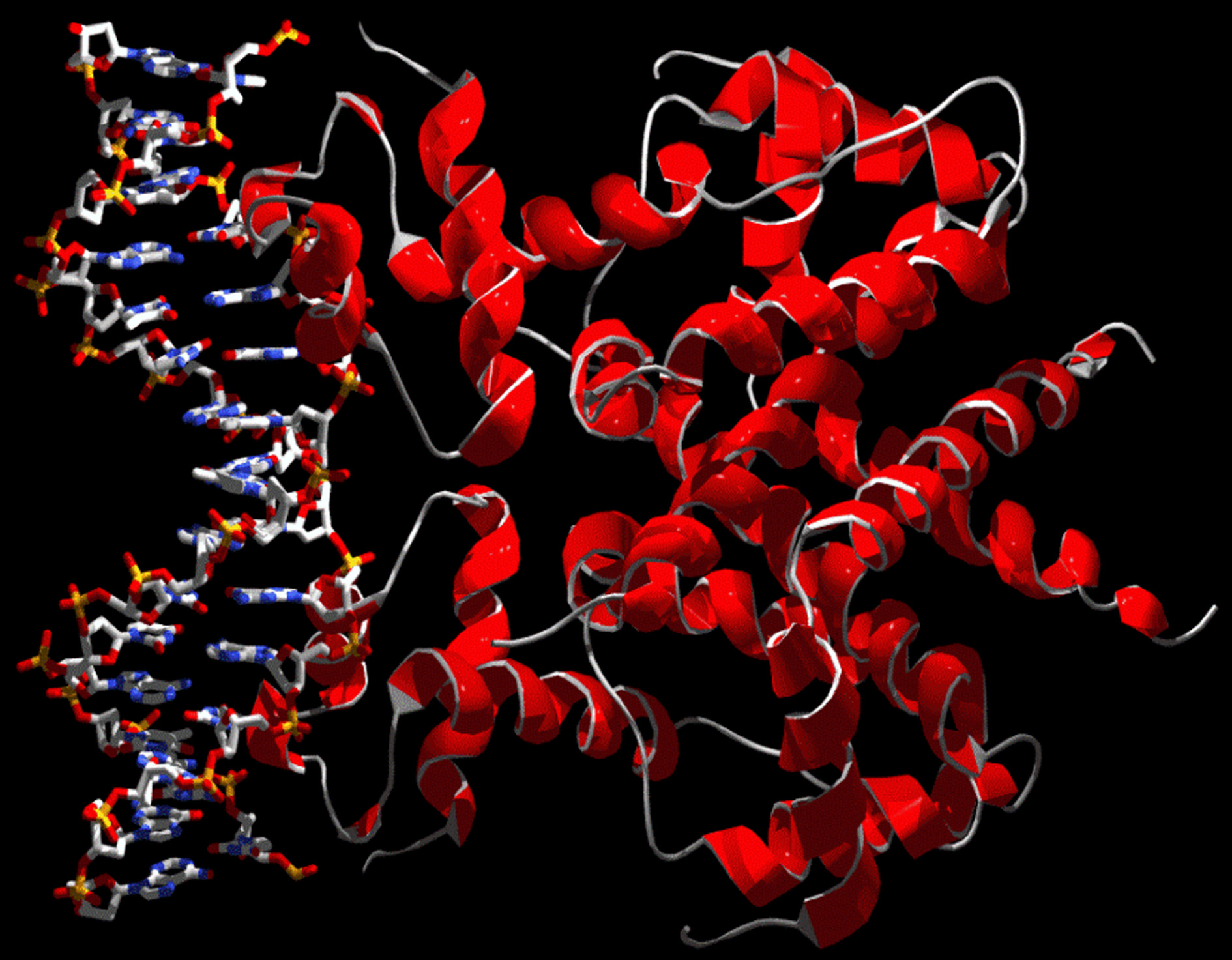
The Chemistry of Signal Transduction in the TetR System
Apart from the therapeutic use of tetracycline derivatives (doxycycline is the most com-monly used tetracycline and a new derivative, tigecycline, was introduced recently), theimportance of the tetracycline/TetR system lies in its use as a ‘‘gene switch'' [4]. TetR can beintroduced into both prokaryotes and eukaryotes in order to be able to turn specific genes onand off at will by administering tetracyclines. TetR has thus become an important tool inmolecular biology.
The mode of action and mechanism of induction of TetR are therefore important, both fordeveloping more specific TetR switches that can be used in parallel in one organism and alsobecause of the general significance of signal transduction in biological processes. Quitegenerally, repressor proteins that switch the expression of other proteins on and off bindto the promoter region of a gene and in doing so prevent expression of the encoded proteinby blocking access of RNA-polymerase, the enzyme that synthesizes transfer RNA based onthe sequence of the gene. Switching occurs when the repressor protein undergoes an allos-teric rearrangement that weakens its binding to the promoter, so that the RNA-polymerasecan displace it and proceed to transcribe the gene. In bacteria, TetR binds to the promotersbelonging to the genes that encode the tetracycline antiporter (TetA), a membrane-boundprotein that pumps tetracycline as the complex of its anion with Mg2+ out of the cell. TetR,however, also regulates its own expression, so that when TetA has pumped all the tetracy-cline out of the cell, more TetR is available to turn off the expression of the two proteinsonce more. Thus, any disadvantages that the bacterium may suffer from the presence of TetAin its cell wall are avoided by the switching mechanism.
The structure of TetR is shown schematically in Figure 1 and its complex with DNA [5] inFigure 2.
Figure 2. The homodimeric tetracycline repressor bound to DNA (structure takenfrom PDB entry 1QPI [5])
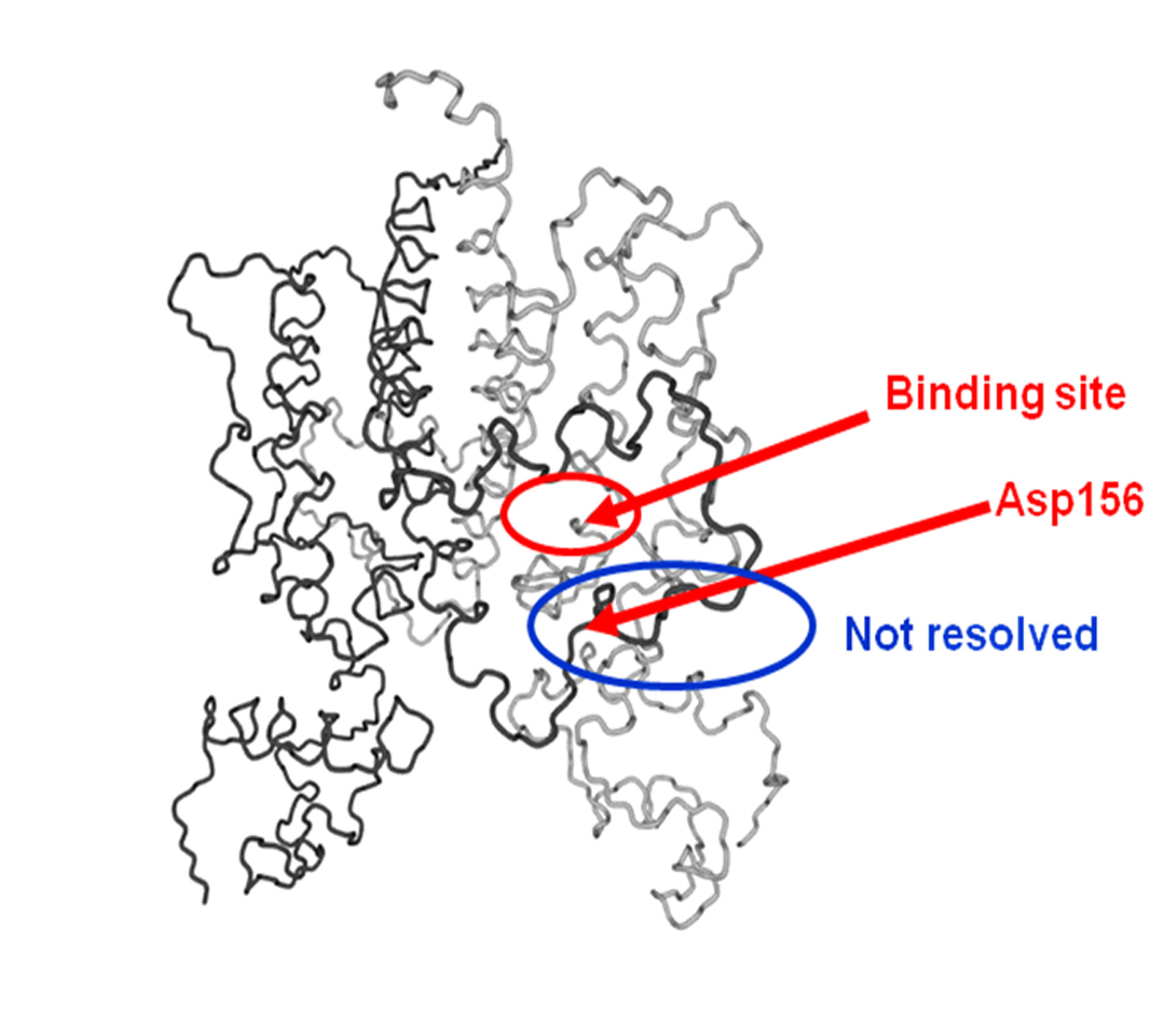
Lanig, H. and Clark, T.
The contact between the protein and DNA occurs via the helices a3, so that the distancebetween the centers of gravity of the Ca atoms of these two helices (denoted R(a3-a3') inthe following) determines whether the repressor fits into the major groove of the doublehelix, and therefore how well it binds.
Molecular Dynamics Simulations
The mechanism of induction of TetR was investigated using molecular dynamics (MD)simulations. In this technique, a classical force field (in this case AMBER Parm94 [6],Gromos87 [7] or OPLS_AA [8]) has been used to simulate the movements of the proteinby solving Newton's second law of motion starting from random velocities for the atoms [9].
Because the TetR protein is very flexible, implicit water solvent must be used and long-range electrostatic interactions included using the particle-mesh Ewald (PME) technique[10]. The details of the simulations are given in the original article [11].
The main problem in determining the mechanism of induction, which was thought to occuron a timescale of microseconds to milliseconds, was that the length of time that could besimulated when the original studies were carried out was limited to tens of nanoseconds.
Thus, it was impossible to observe the allosteric change on induction in the simulations.
However, facile rearrangements of biological macromolecules reveal themselves in thenormal vibrations. The normal modes with the lowest frequency and the largest amplitudeare usually those that lead to the rearrangement. It is not necessary to calculate the force-constant matrix for the system as the normal modes can be calculated by diagonalizing themass-weighted covariance matrix obtained from an MD simulation [12].
Figure 3. Ca trace of the backbone of TetR showing the flexible loop, which is notresolved in most X-ray structures, the tetracycline binding site and the position ofAsp156.
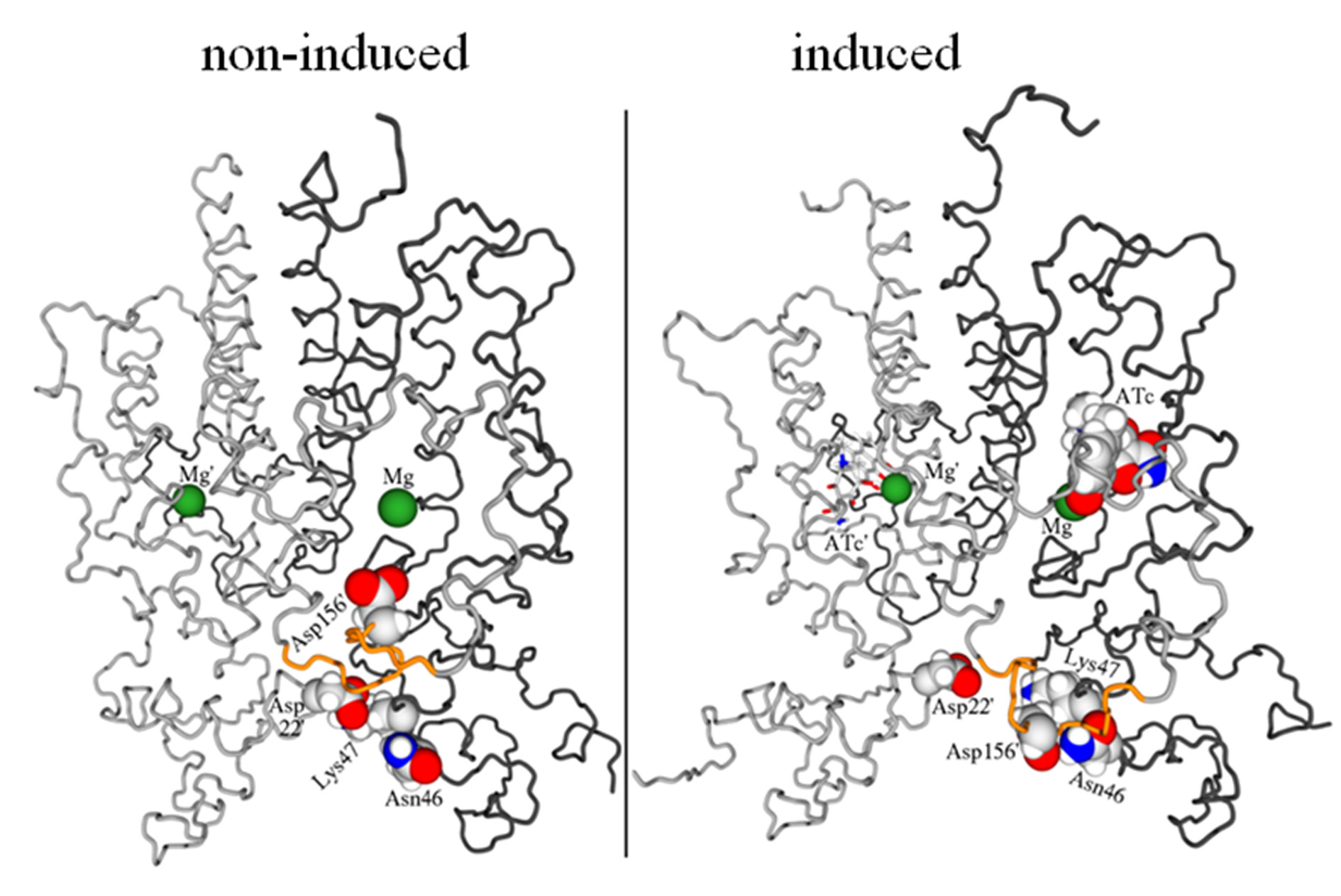
The Chemistry of Signal Transduction in the TetR System
Figure 3 shows the main features of the induction mechanism revealed by the lowest-energynormal mode. The so-called flexible loop, indicated by the blue oval in Figure 3, has onlyrecently been resolved in some X-ray structures. The lowest-energy normal mode revealed avery large movement of aspartate 156 away from the tetracycline binding site towards theDNA-binding heads. This movement is the key to the induction mechanism, which is shownschematically in Figure 4.
Figure 4. Schematic view of the mechanism of induction of TetR by tetracyclinescomplexed to magnesium. [11] In the non-induced structure (left), Asp156 forms aloose salt bridge to the magnesium ion in the tetracycline binding site and Asp22forms a tight salt bridge with Lys47 to bind the two DNA-binding heads together. Inthe induced form (right), ATc displaces Asp156 from the magnesium. The aspartatethen migrates towards the DNA-binding heads and forms a new salt bridge withLys47, thus breaking the one with Asp22. This removes the salt bridge between thetwo binding heads and allows them to move apart. The flexible loop is highlighted inyellow.
The effect of the inducer (in this case ATc) is to displace Asp156 from the magnesium ion.
The aspartate then swings down towards the DNA-binding heads as part of the large move-ment of the flexible loop observed in the lowest-frequency normal mode. It displaces Lys47from its salt bridge with Asp22 and thus removes the salt bridge that binds the two DNA-binding heads together.
The Reverse Phenotype
One of the most fascinating aspects of TetR is that a single mutation is enough to reverse thebehavior of the repressor [13]. The reverse phenotype is produced, for instance, by mutatingGly95 in the wild type TetR class BD to glutamate. We will designate this mutant revTetR.
RevTetR is induced in the absence of tetracyclines, but not in their presence. Its inductionbehavior is thus exactly the opposite of the wild type. We have also performed long MD
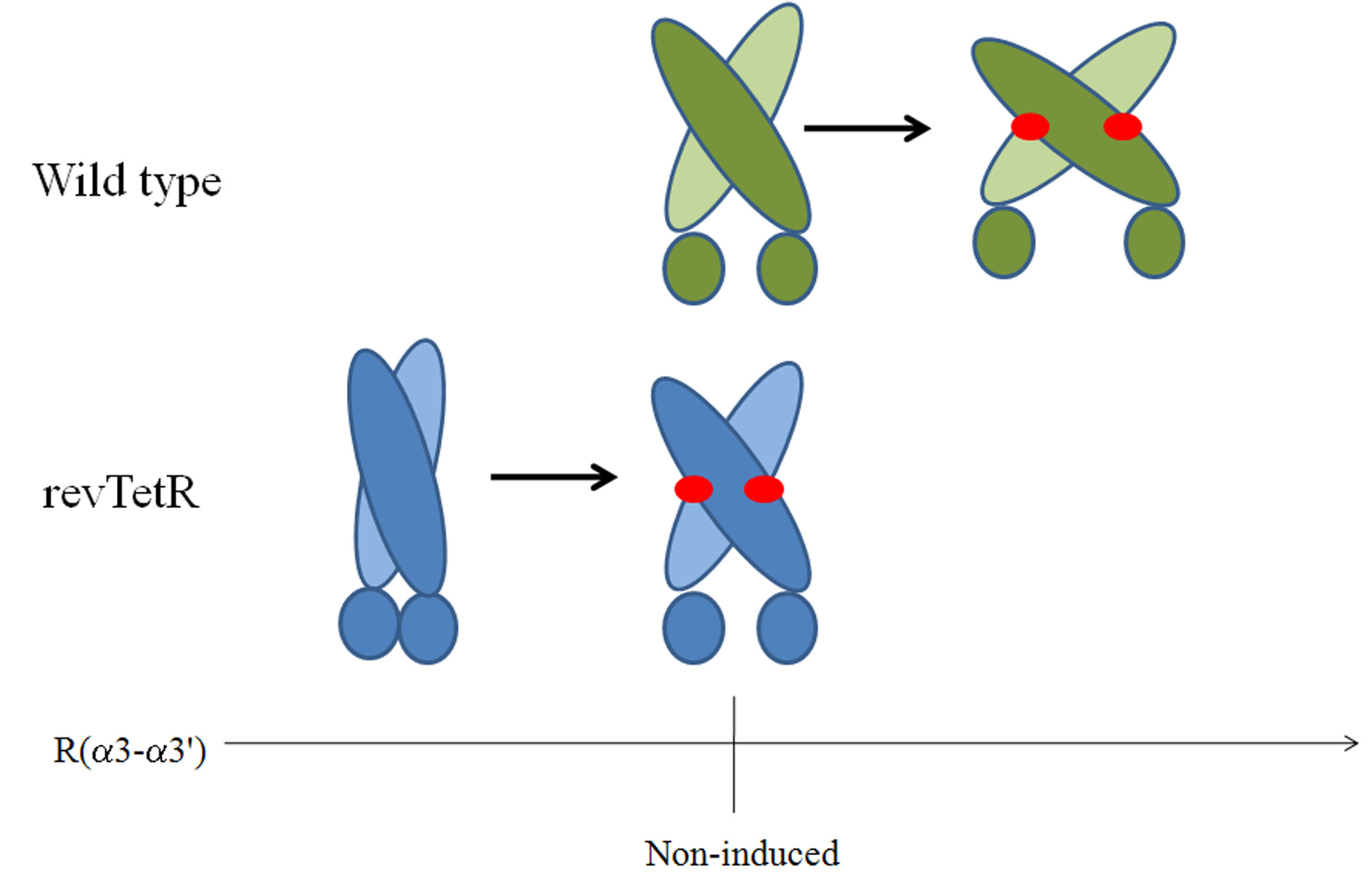
Lanig, H. and Clark, T.
simulations on this mutant [14] and conclude that the mechanism of induction is exactly thesame as that observed for wild type TetR. However, the structure of the revTetR dimerwithout tetracycline differs significantly from that of the wild type.
Figure 5. Schematic diagram of the mechanism of induction of the TetR wild type(green above) and the reverse phenotype (blue, below). The horizontal axis representsthe distance between the DNA-binding heads, which is optimal for binding to DNA atthe distance marked ‘‘Non-induced''. Docked tetracycline molecules are indicated asred ovals.
Figure 5 shows the situation schematically. The R(a3-a3') distance is too short to bindeffectively to DNA in the wild type without tetracycline. The induction movement is thesame in the two TetR variants, but in the wild type it extends R(a3-a3') to a value too largeto to bind ideally to DNA, whereas in revTetR it increases R(a3-a3') to exactly the valueneeded for optimal binding. Note, however, that revTetR shows signs of denaturing far moreeasily than the wild type and is stabilized by tetracyclines [15], so that denaturation (andhence induction) may occur in the absence of tetracyclines. Such an effect is too slow to berevealed by the MD simulations.
Detecting Induction
Experimental determinations of the induction state of TetR are time-consuming and difficult.
It would therefore be useful to be able to determine whether a given TetR variant or mutationis induced in the presence of a given inducer. The R(a3-a3') criterion suggested above may,however, indicate induction in X-ray structures or those taken from MD simulations. In thefollowing, we examine these two possibilities.
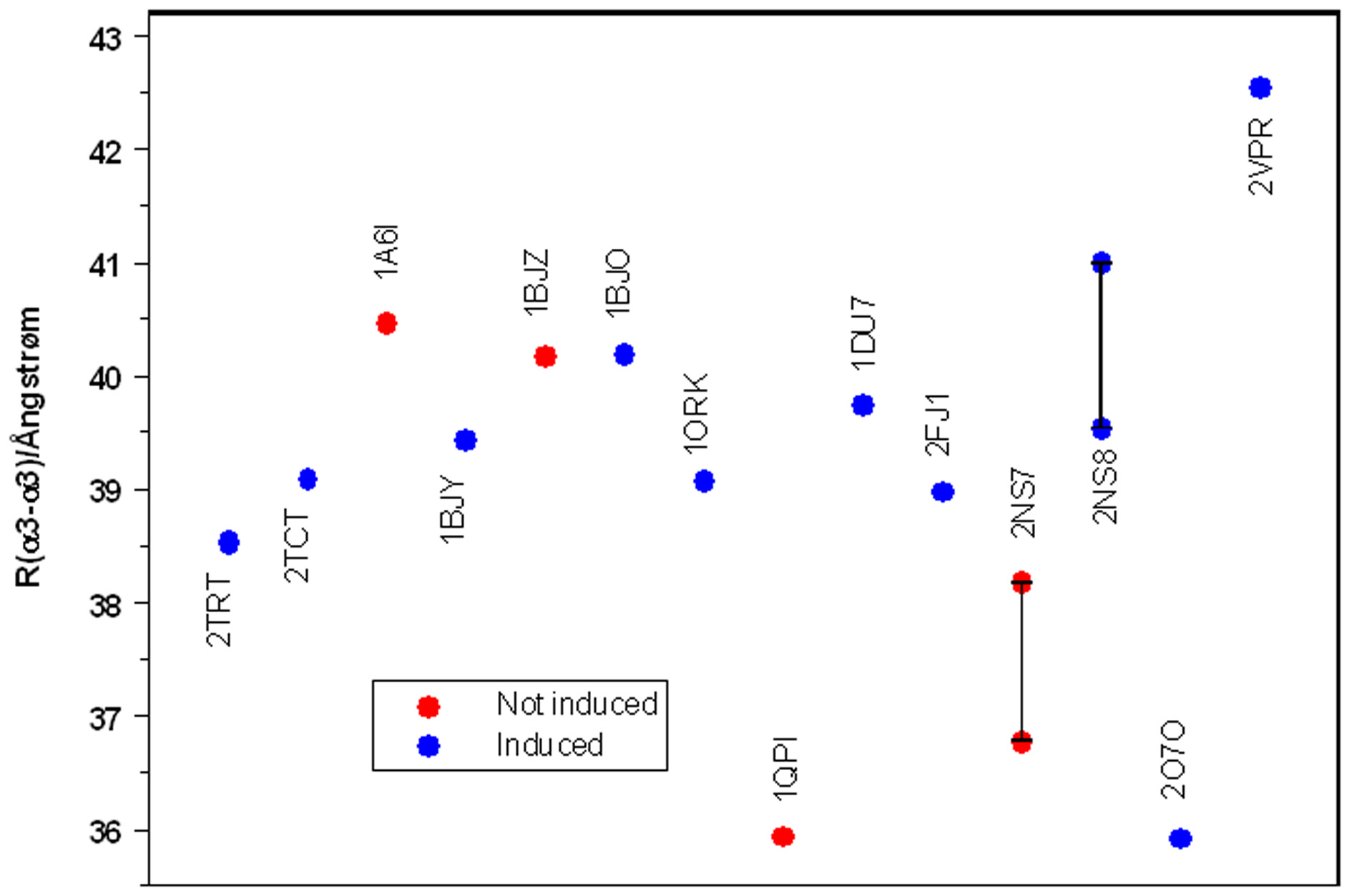
The Chemistry of Signal Transduction in the TetR System
Determining Induction from X-ray Structures
A total of 14 X-ray structures of TetR have been published [16 – 24] with and without avariety of inducers and in one case complexed to DNA. One additional TetR class Dstructure (2VKE) complexed with Tc and Co2+ was published 2007 and shows almost nodifferences in backbone geometry and ligand position when comparing to 2TRT. Figure 6shows a plot (in chronological order) of the R(a3-a3') distances obtained from thesestructures. There are actually 16 distances because the structures 2NS 7 and 2NS 8 containtwo different TetR dimers in the unit cell. The points in the graph are color coded accordingto whether TetR is induced or not in the combination found in the X-Ray structure.
Figure 6. Plot of the R(a3-a3') distances obtained from TetR X-ray structures. Thelabels for the points indicate the PDB-codes. Two values are given for 2NS 7 and2NS 8 because there are two non-equivalent TetR dimers in their unit cells. The pointsare plotted in the order of their publication.
Figure 6 does not reveal a convincing relationship between R(a3-a3') and the state ofinduction of TetR. The definitive R(a3-a3') value for the non-induced state of TetR is thatfor 1QPI, which is the X-ray structure in which the TetR dimer is bound to DNA. However,this distance is almost identical to that found in structure 2O7O, a complex with the stronginducer doxycycline. Quite generally, the R(a3-a3') values from the X-ray structures appearnot to be related to the induction state of TetR.
This is not completely surprising. Signal-transduction proteins that switch by undergoing anallosteric change are by their nature very flexible. It is therefore reasonable to expect that theforces needed to switch the conformation are of the same order of magnitude as
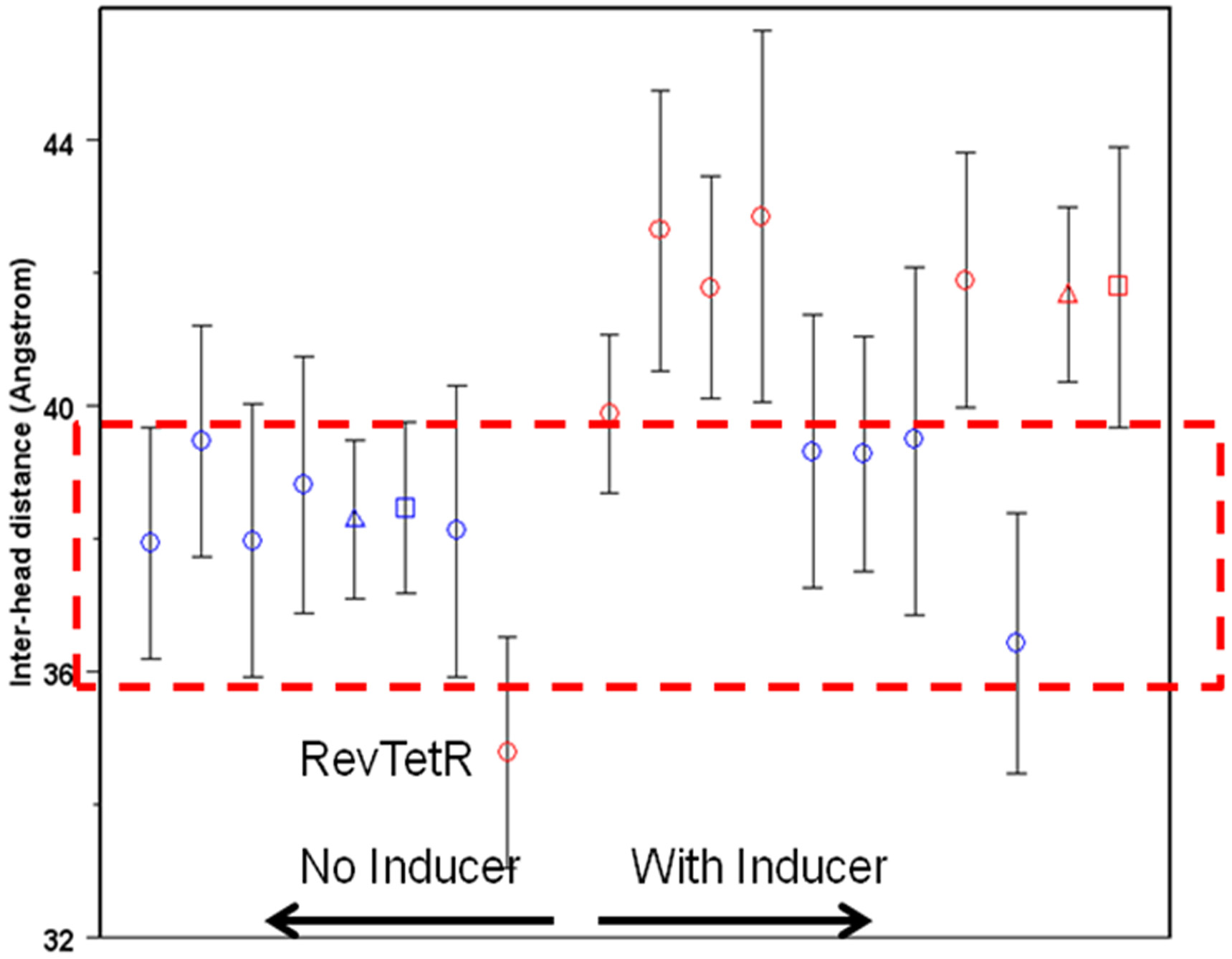
Lanig, H. and Clark, T.
crystal-packing forces. The structures of signal-transduction proteins in crystals will there-fore be perturbed quite strongly from their solution conformations and cannot be expected toreproduce the induction state of the protein correctly. Note, for instance, that if the first fiveX-ray structures of TetR had been used to propose a mechanism, we would have concludedthat the R(a3-a3') distance in the non-induced form is larger than in the induced one – thereverse of what we now believe.
Determining Induction from MD Simulations
Molecular dynamics simulations offer a possible alternative for determining the inductionstate of TetR. However, for this to be the case, the allosteric rearrangement must take placeon a time scale that makes it probable within the length of a simulation, which in our case is50 – 100 ns. We will show below that, contrary to our expectations, this is the case.
Figure 7. Plot of the R(a3-a3') distances obtained from TetR MD simulations. Redpoints indicate those that are experimentally induced, blue those that are not. Theshapes of the symbols indicate the force field used. Circles are AMBER Parm94, [6]triangles Gromos87 [7] and squares OPLS_AA. [8] The dashed box indicates thelimits of R(a3-a3') for non-induced TetR. The error bars represent ± one standarddeviation over the sampling period.
Figure 7 shows the results of 19 simulations, all of which were 50 ns or longer. In contrast tothe X-ray results, the R(a3-a3') distances from the simulations show a clear relationship tothe induction state of TetR. All simulations for which R(a3-a3') falls within the ‘‘non-induction window'' from approximately 36 – 40 A
˚ represent TetR systems that are found
experimentally not to be induced. Those above and below this window are induced.
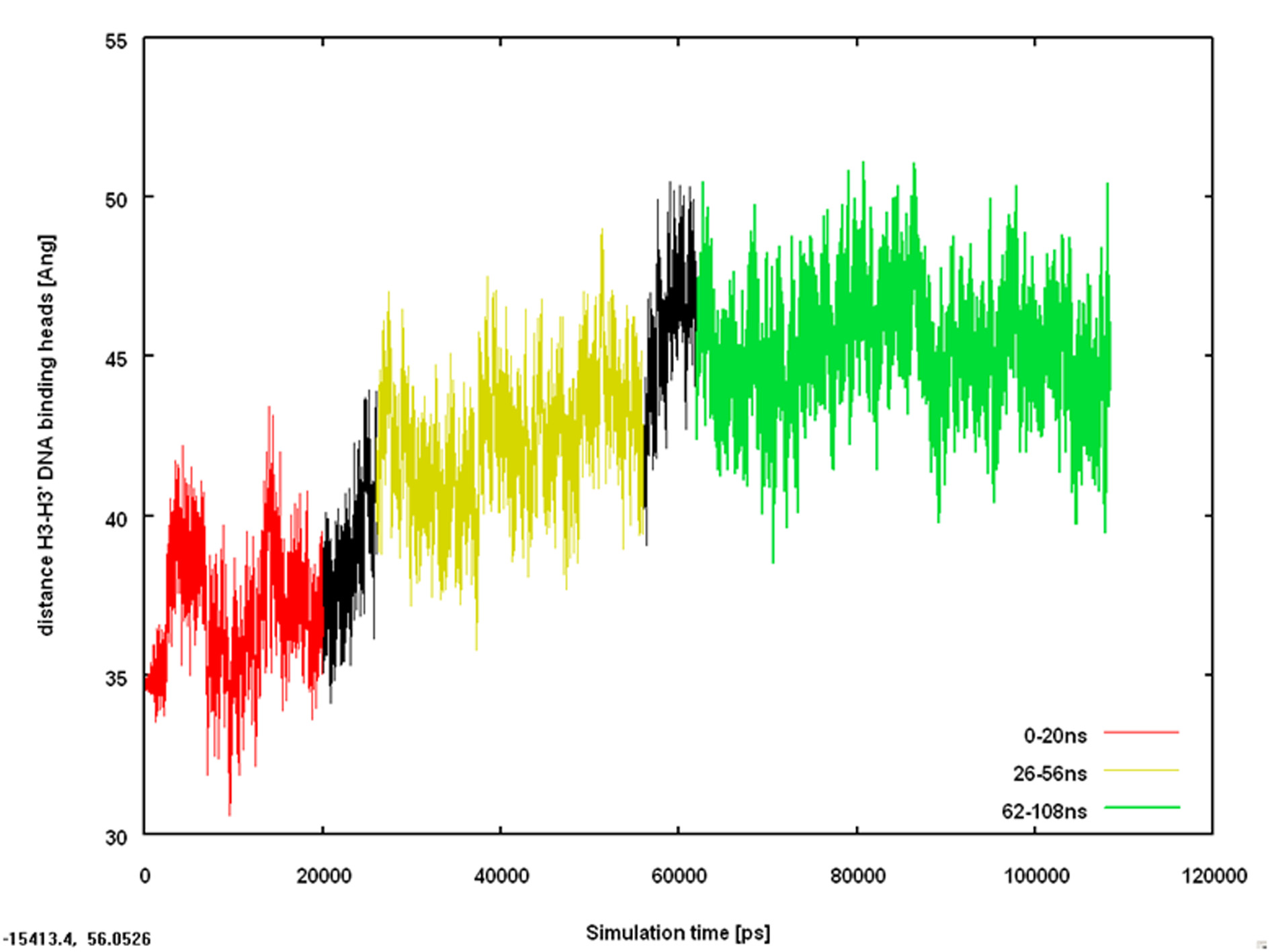
The Chemistry of Signal Transduction in the TetR System
The differences between some simulations close to the borders are not statistically signifi-cant, but nevertheless the simulations provide a remarkably reliable prediction of the induc-tion state.
This result is quite remarkable. MD simulations with three different force fields on a varietyof combinations of TetR variants and inducers can reproduce the induction behavior of TetRwith far higher reliability than X-ray structures, which are perturbed too much to reflect theinduction state correctly. This result even appears to be fairly independent of the force fieldused. The simulations used three different common force fields, AMBER Parm94, [6]Gromos87 [7] and OPLS_AA [8]. However, many of the simulations did not start in theregion of the mean R(a3-a3') values over the sampling periods. The R(a3-a3') valuesshown in Figure 7 are taken from the later stages (typically after 20 ns or longer) of thesimulations after TetR has relaxed to its preferred geometry under the conditions of thesimulation. This process in itself is of interest.
Allosteric Rearrangements in MD Simulations
Figure 8 shows a trace of the R(a3-a3') distance in an AMBER Parm94 simulation of TetRwith doxycycline starting from the geometry of the 2O7O X-ray structure. This is thestructure that exhibits the same R(a3-a3') distance as 1QPI, the complex with DNA.
Figure 8. Trace of the R(a3-a3') distance against time for a simulation of TetR withtwo doxycycline molecules starting from the 2O7O X-ray structure.
Lanig, H. and Clark, T.
The red region of the plot from 0 – 20 ns has a mean R(a3-a3') distance of 36.9 A
shows oscillations that appear to increase in amplitude with time. Between 20 and 62 ns,R(a3-a3') increases steadily to a final value (green region, 62 – 108 ns, mean R(a3-a3') = 45.3 A
˚ ) in which R(a3-a3') no longer exhibits the strong oscillations seen at the
beginning of the simulation. Thus, the simulation remains stable at a non-induced value ofR(a3-a3') for the first 20 ns before undergoing a transition over 40 ns to achieve a final,apparently stable, induced conformation. We emphasize that this process does not necessa-rily correspond to a real induction event in the biological system, but strictly speaking onlyto the relaxation of the strained 2O7O X-ray structure. Nevertheless, the fact that the initialconformation remains stable for 20 ns (the complete length of many simulations reported inthe current literature) is very significant. This is as far as we are aware the first directobservation in an MD simulation of the pre-equilibrium mechanism of induction [25]. Inthis mechanism, which has found wide acceptance, the inducer first docks into the non-induced conformation of the repressor to form a metastable complex, which eventuallyrelaxes to the induced conformation of the repressor-inducer complex. In the reverse pro-cess, the inducer dissociates from the induced complex to form a metastable, uncomplexedinduced conformation of the repressor, which then can relax to the stable non-inducedconformation.
We have shown that MD simulations of 50 – 100 ns are an effective tool for identifying themechanism of induction of TetR and for detecting its state of induction. These conclusionsare presumably also valid for other signal-transduction proteins that vary their bindingaffinity by an allosteric rearrangement. Perhaps predictably, X-ray structures are not wellsuited for determining the induction state of signal-transduction proteins because we believethe crystal-packing forces to be of the same order of magnitude as those that cause theallosteric rearrangement.
It is perhaps misleading to discuss ‘‘the mechanism of induction''. Figure 9 illustrates thispoint.
Thus, not induction is unique, but rather the fact that signal-transduction proteins can bind sostrongly to the promoter and that this bound conformation is so robust to environmentalfactors such as temperature, pH, ion concentrations etc. that should not be able to causeinduction. Induction can be caused by just about any structural change, including denatura-tion, that lowers the binding affinity. Thus, one and the same signal-transduction proteinmay be induced in many ways. This is in fact true of TetR, which is induced by tetracyclinesin the presence of magnesium, by 5a,6-anhydrotetracycline [26] and the TetR-inducingprotein TIP [22] in its absence. In the latter case, a different mechanism of induction isobserved to that found with tetracycline-magnesium complexes [27].
The Chemistry of Signal Transduction in the TetR System
Figure 9. Schematic illustration of the induction of a signal-transduction protein. Thesmall, specific red area represents the non-induced protein, which can bind strongly tothe promoter. The surrounding yellow area represents induced geometries that do notbind as strongly. Clearly, there are very many ways to distort the protein so that it nolonger binds strongly.
This work has been supported for the last eight years by the Deutsche Forschungsge-meinschaft as part of SFB 473, Mechanisms of Transcriptional Regulation. We thankWolfgang Hillen, Chris Berens and Peter Gmeiner for many enlightening discussions. Noneof the results described here would have been obtained without the support of FrankBeierlein, Olaf Othersen, Ute Seidel and Florian Haberl. We gratefully acknowledge largeallocations of computer time on HLRB II.
Lanig, H. and Clark, T.
Saenger, W., Orth, P., Kisker, C., Hillen, W., Hinrichs, W. (2000) The TetracyclineRepressor-A
39:2042 – 2052.
Walsh, C. (2003) Antibiotics: Actions, Origins, Resistance. ASM Press, Washington,D.C.
Scholz, O., Schubert, P., Kintrup, M., Hillen, W. (2000) Tet Repressor Inductionwithout Mg2+. Biochemistry 39:10914 – 10920.
Berens, C., Hillen, W. (2003) Gene regulation by tetracyclines. Constraints of resis-tance regulation in bacteria shape TetR for application in eukaryotes. FEBS Journal270:3109 – 3121.
Orth, P., Schnappinger, D., Hillen, W., Saenger, W., Hinrichs, W. (2000) Structuralbasis of gene regulation by the tetracycline inducible Tet repressor-operator system.
Nature Struct. Biol. 7:215 – 219.
Cornell, W.D., Cieplak, P. Bayly, C. I., Gould, I.R., Merz, K.M. Jr., Ferguson, D.M.,Spellmeyer, D.C., Fox, T., Caldwell, J.W., Kollman, P.A. (1995) A Second Genera-tion Force Field for the Simulation of Proteins, Nucleic Acids, and Organic Mole-cules. J. Am. Chem. Soc. 117:5179 – 5197.
Van Gunsteren, W.F., Berendsen, H.J.C. (1987) Groningen Molecular SimulationLibrary, Groningen.
Jorgensen, W.L., Maxwell, D.S., Tirado-Rives, J. (1996) Development and Testing ofthe OPLS All-Atom Force Field on Conformational Energetics and Properties ofOrganic Liquids. J. Am. Chem. Soc. 118:11225 – 11236.
Allen, M.P., Tildesley, D.J. (1987) Computer Simulation of Liquids, Oxford Univer-sity Press, Oxford.
Darden, T., York, D., Pedersen, L. (1993) Particle mesh Ewald: An N·log(N) methodfor Ewald sums in large systems. J. Chem. Phys. 98:10089 – 10092.
Lanig, H., Othersen, O.G., Beierlein, F. R., Seidel, U., Clark, T. (2006) MolecularDynamics Simulations of the Tetracycline-Repressor Protein: The Mechanism ofInduction. J. Mol. Biol. 359:1125 – 1136.
Amadei, A., Linssen, A.B.M., Berendsen, H.J.C. (1993) Essential Dynamics ofProteins. Proteins: Struct. Funct. Genet. 17:412 – 425.
The Chemistry of Signal Transduction in the TetR System
Scholz, O., Henßler, E.-M., Bail, J., Schubert, P., Bogdanska-Urbaniak, J., Sopp, S.,Reich, M., Wisshak, S., Ko¨stner, M., Bertram, R., Hillen, W. (2004) Activity reversalof Tet repressor caused by single amino acid exchanges. Mol. Microbiol.
53:777 – 789.
Seidel, U., Othersen, O.G., Haberl, F., Lanig, H., Beierlein, F.R., Clark, T. (2007)Molecular Dynamics Characterization of the Structures and Induction Mechanisms ofa Reverse Phenotype of the Tetracycline Receptor. J. Phys. Chem. B 111:6006 – 6014.
Resch, M., Striegl, H., Hennsler, E.M., Sevvana, M., Egerer-Sieber, C., Schiltz, E.,Hillen, W., Muller, Y.A. (2008) A protein functional leap: how a single mutationreverses the function of the transcription regulator TetR. Nucleic Acids Res.
36:4390 – 4401.
Hinrichs, W., Kisker, C., Du¨vel, M., Mu¨ller, A., Tovar, K., Hillen, W., Saenger, W.
(1994) Structure of the Tet Repressor-Tetracycline Complex and Regulation of Anti-biotic Resistance. Science 264:418 – 420.
Kisker, C., Hinrichs, W., Tovar, K., Hillen, W., Saenger, W. (1995) The ComplexFormed Between Tet Repressor and Tetracycline-Mg2+ Reveals Mechanism of Anti-biotic Resistance. J. Mol. Biol. 247:260 – 280.
Orth, P., Cordes, F., Schnappinger, D., Hillen, W., Saenger, W., Hinrichs, W. (1998)Conformational Changes of the Tet Repressor Induced by Tetracycline Trapping.
J. Mol. Biol. 279:439 – 447.
Orth, P., Saenger, W., Hinrichs, W. (1999) Tetracycline-Chelated Mg2+ Ion InitiatesHelix Unwinding in Tet Repressor Induction. Biochemistry 38:191 – 198.
Orth, P., Schnappinger, D., Sum, P.-E., Ellestad, G. A., Hillen, W., Saenger, W.,Hinrichs, W. (1999) Crystal Structure of the Tet Repressor in Complex with a NovelTetracycline, 9-(N, N-dimethylglycylamido)-6-demethyl-6-deoxy-tetracycline. J. Mol.
Biol. 285:455 – 461.
Orth, P., Schnappinger, D., Hillen, W., Saenger, W., Hinrichs, W. (2000) Structuralbasis of gene regulation by the tetracycline inducible Tet repressor-operator system.
Nature Struct. Biol. 7:215 – 219.
Luckner, S.R., Klotzsche, M., Berens, C., Hillen, W., Muller, Y.A. (2007) How anAgonist Peptide Mimics the Antibiotic Tetracycline to Induce Tet-Repressor. J. Mol.
Biol. 368:780 – 790.
Aleksandrov, A., Proft, J., Hinrichs, W., Simonson, T. (2007) Protonation Patterns inTetracycline:Tet Repressor Recognition: Simulations and Experiments. ChemBio-Chem 8:675 – 685.
Lanig, H. and Clark, T.
Aleksandrov, A., Schuldt, L., Hinrichs, W., Simonson, T. (2008) Tet RepressorInduction by Tetracycline: A Molecular Dynamics, Continuum Electrostatics, andCrystallographic Study. J. Mol. Biol. 378:896 – 910.
Kern, D., Zuiderweg, E.R.P. (2003) The role of dynamics in allosteric regulation.
Curr. Opin. Struct. Biol. 13:748 – 757.
Scholz, O., Schubert, P., Kintrup, M., Hillen, W. (2000) Tet Repressor Inductionwithout Mg2+. Biochemistry 39:10914 – 10920.
Haberl, F. (2008) PhD thesis, Universita¨t Erlangen-Nu¨rnberg.
Source: http://www.beilstein-institut.de/download/629/10_clark.pdf
EL PROCEDIMIENTO DE SELECCION DEL CONTRATISTA* (Segunda parte) Dr. Rodolfo Carlos Barra 74.- IMPUGNABlLIDAD DE LOS ACTOS DEL PROCEDIMIENTO En supra, Cap. IV, parág. 23 se desarrolló, al analizar el elemento "procedi- miento" como requisito esencial del contrato administrativo. la doctrina de los "actos coligados" como característica fundamental del procedimiento contractual
The Ties That Bound a County Railway Heritage Tour of Perth County Railway rivalry, expansions and an even an explosion created drama in Stratford and Perth County long before any actor set foot on a stage. Experience the railway heritage of Stratford, St. Marys and Listowel while hiking along former railway lines, learning about the interesting people who helped build the railway's strong hold and viewing some of the last, and best preserved railway buildings in Ontario.