Biodegradation of potentially harmful compounds in wastewater treatment
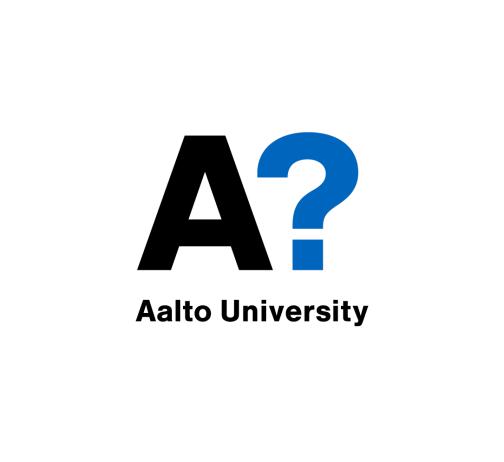
Aalto University School of Engineering
Pia Ahlgren
BIODEGRADATION OF POTENTIALLY HARMFUL COMPOUNDS IN
WASTEWATER TREATMENT
Master's Thesis submitted for examination for the degree of Master of Science in Technology. Espoo 25.4.2012 Supervisor: Professor Riku Vahala Instructor: Lic.Sc.(Tech.) Pirjo Rantanen
AALTO UNIVERSITY
ABSTRACT OF THE MASTER'S THESIS
SCHOOLS OF TECHNOLOGY PO Box 11000, FI-00076 AALTO http://www.aalto.fi
Author: Pia Ahlgren
Title: Biodegradation of potentially harmful compounds in wastewater treatment
School: School of Engineering
Department: Civil and Environmental Engineering
Professorship: Water and Wastewater Engineering
Supervisor: Professor Riku Vahala
Instructor: Lic.Sc.(Tech.) Pirjo Rantanen
To study Potentially Harmful Compounds (PHCs) in the environment and their fate in Wastewater Treatment Plants (WWTPs) is a growing area of interest among researchers. Persistent Organic Pollutants (POPs) and Endocrine Disruptive Compounds (EDCs) are a concern together with Pharmaceuticals and Personal Care Products (PPCP). While advanced treatments are being considered, the fate of compounds in activated sludge is important to understand. Biodegradation is the only way of entirely removing PHCs in WWTPs and stopping them from ending up in the environment.
In this thesis a laboratory scale Sequencing Batch Reactor (SBRs) with four parallel reactors was built and the operation optimized. Sludge from the Suomenoja WWTP was used to start the reactors and they were then fed with synthetic wastewater. The operational conditions were chosen to be typical values in Finnish WWTPs. A marker compound, ibuprofen, was chosen to be studied in the reactors. The objective was to verify laboratory procedures and obtain a primary biodegradation rate and biodegradation rate constant of the compound. Ibuprofen is a non-steroidal acidic anti-inflammatory drug that is largely used in Finland and found in environmental waters as well as in WWTPs.
The synthetic wastewater was spiked with ibuprofen on four occasions. Samples from the reactors were taken at different times during aeration to analyze the ibuprofen concentration. This way the biodegradation rate and biodegradation rate constant (kbiol) was determined for ibuprofen in the SBRs. The average biodegradation of ibuprofen in the reactors was 30-50%. A kbiol value of 1.9l/gSS/d was obtained. The values obtained in the study were in good agreement with previous studies. The slightly lower value for the constant could possibly be explained by the high F/M-ratio. Further research on the biodegradation mechanisms and the effect of different treatment conditions are needed to optimize the removal of ibuprofen. Also the accuracy of the analyses needs to be improved.
Language: English
Number of pages: 14+91+10
Keywords: biodegradation, ibuprofen, PHC, SBR, wastewater treatment,
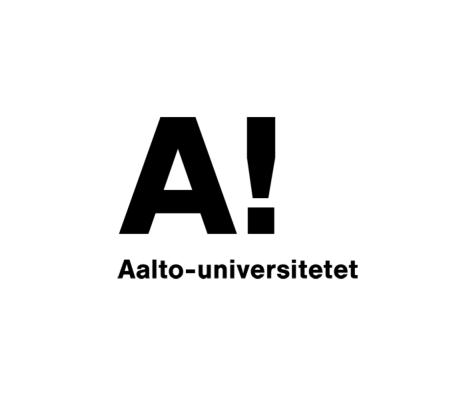
AALTO-UNIVERSITETET
SAMMANDRAG AV DIPLOMARBETE
HÖGSKOLORNA FÖR TEKNIK PB 11000, FI-00076 AALTO http://www.aalto.fi
Författare: Pia Ahlgren
Titel: Biologisk nedbrytning av potentiellt skadliga föreningar i avloppsrening
Högskola: Högskolan för Ingenjörsvetenskaper
Institution: Institutionen för samhälls- och miljöteknik
Professur: Vatten- och avloppsteknik
Övervakare: professor Riku Vahala
Handledare: TkL Pirjo Rantanen
Att studera potentiellt skadliga föreningar i naturen och i avloppsreningsverk har under senaste åren blivit en prioritet bland forskare. Långlivade organiska föreningar, så kallade POPs, föreningar som stör det endokrina systemet (EDCs), läkemedel och ämnen i hygienprodukter och kosmetika är ett växande bekymmer. Att studera dessa ämnens öde i avloppsreningsverk, speciellt aktivslamprocessen, är viktigt före man beslutar ifall mer avancerade reningsmetoder är nödvändiga att implementera. Biologisk nedbrytning är det enda sättet att totalt eliminera potentiellt skadliga föreningar i avloppsreningsverk och hindra dem ifrån att hamna i naturen. I detta diplomarbete byggdes ett satsvis biologiskt reningsverk (SBR) i laboratorieskala med fyra parallellreaktorer och deras funktion optimerades. Processen startades med slam från Finno avloppsreningsverk varefter reaktorerna matades med syntetiskt avloppsvatten. Förhållanden, där reaktorerna opererade, var valda för att bäst representera förhållanden i finska avloppsreningsverk. En förening, ibuprofen, valdes för att fungera som markör och studerades i processen. Syftet var att verifiera provtagningsmetoderna i laboratoriet och få preliminära värden för biologisk nedbrytning av ibuprofen. Ibuprofen är en icke-steroid antiinflammatorisk medicin som används allmänt och som sedan har hittats i vattendrag och i avloppsreningsverk i Finland. Ibuprofen tillsattes i det syntetiska avloppsvattnet fyra gånger under studieperioden. Sampel från reaktorerna togs vid olika tider under luftningsfasen för att sedan kunna bestämma den biologiska nedbrytningen och nedbrytningskoefficienten. Ibuprofen nedbröts i medel 30-50% och därav kunde en biologisk nedbrytningskoefficient (kbiol) på 1,9l/gSS/d bestämmas. Dessa värden stämmer bra överens med värden från tidigare studier. Att nedbrytningskoefficienten dock var lite lägre än i andra studier, kan ha berott på den höga slambelastningen. Fortsatta studier på mekanismer bakom biologisk nedbrytning av ibuprofen behövs för att ytterligare optimera dess eliminering i avloppsreningsverk. Det krävs även bättre analysnoggrannhet för att erhålla exakta värden på nedbrytningen.
Datum: 25.4.2012
Språk: Engelska
Sidantal: 14+91+10
Nyckelord: Avloppsrening, biologisk nedbrytning, ibuprofen, SBR, skadliga föreningar
This study was made in the Water Engineering research group of the Civil Engineering
Department. It was a pre-study for future research to be made in the Water Engineering
research group. The study was funded by Maa- ja Vesitekniikan Tuki ry and their
contribution is greatly appreciated.
First I would like to thank prof. Riku Vahala and Lic.Sc.(Tech.) Pirjo Rantanen for
making this thesis possible. The advice and support I got from Pirjo during the whole
process was invaluable. A special thank go to the entire staff at the Water Laboratory,
especially to Aino Peltola and Ari Järvinen for helping me during the experiments. I
would also like to thank Dr Michela Mulas and Lic.Sc.(Tech.) Henri Haimi for
providing me with tips and materials for my work.
All of this would not have been possible without the support from my family. Thanks go
also to my friends who provided me with a memorable time as student and much needed
relief from my studies. Lastly I would also like to express my gratitude to Tomi for his
patience and support.
Contents
Literature part
Experimental Part
Appendix I Results from analyses
Appendix II Planning and operating a pilot scale Sequencing Batch Reactor (SBR)
Appendix III CD with pictures of the Sequencing Batch Reactor
Symbols and legends
Biological Oxygen Demand
Chemical Oxygen Demand
Food per Mass Ratio
g BOD/ g MLSS /dg COD/ g MLSS/d
Hydraulic Retention Time
Mixed Liquir Suspended Solids
Mixed Liquir Volatile Suspended Solids mass/volume
Sludge Volume Index
Solids Retention Time
Total Phosphorous
Describes the ability to ionize or dissociate to
Dissociation constant
Biodegradation rate constant
Describes the biodegradation potential
Sorption coefficient
Describes the sorption potential
Octanol-water-partition coefficient Describes lipophilicity of a compound
Defined Daily Dose
Endocrine Disruptive Compound
Hexabromocyclododecane
High Performance Liquid Chromatography
Hydraulic Retention Time
Liquid Chromatography
Long-Chain Chlorinated Paraffarin
Membrane Bioreactor
Medium-Chain Chlorinated Paraffarin
Mass Spectrometry
Nonylphenol Ethoxylate
Polycyclic Aromatic Hydrocarbon
Persistent, Bioaccumulative, Toxicity
Prefluorinated Alkyl Compounds
Perfluorooctanoic Acid
Perfluorosulfonate
Potentially Harmful Compound
Persistent Organic Pollutant
Pharmaceuticals and Personal Care Products
Quantitative Structure-Activity Relationship
Returned Activated Sludge
Sequencing Batch Reactor
Short-Chain Chlorinated Paraffarin
Solid Phase Extraction
Tetrabromobisphenol A
Ultra Performance Liquid Chromatography
Volatile Organic Compound
Wastewater Treatment Plant
List of Tables
List of Figures
1. Introduction
1.1 Background
Municipal wastewater has been treated for over a hundred years. In the beginning the
priority was to remove organic materials, suspended solids and pathogens. The
objective of nutrient removal in Wastewater Treatment Plants (WWTPs) was raised,
when it was found that the large quantities of nutrients that are led to environmental
waters increase the growth of algae. The aim was to improve water quality for leisure
purposes. Although these goals have not been met in all wastewater treatment plants, in
the industrialized world the focus has now been shifting towards other Potentially
Harmful Compounds (PHCs) in wastewater. Pharmaceutical residues, pesticides and
compounds that are used in solvents, paints and cleaning agents, are all found in
environmental waters. The biggest source of harmful compounds in drinking water,
rivers, oceans and lakes is believed to be the effluents from WWTPs. These compounds
can be mutagenic, carcinogenic, toxic and hormone disrupters. When released into the
environment, PHCs may harm both organisms living in nature and humans. A danger is
also that the compounds pass into drinking water supplies. It is important to find out
what PHCs are in our waters and how we can eliminate these in wastewater treatment.
The challenge is to detect many different kinds of compounds at low concentrations.
The compounds that have been described in this study are a selection of different
chemicals that lately have raised concerns due to their presence in waters, their toxicity
and/or are being largely used in society. The compounds in this study are found, in
varying degrees, in WWTPs.
1.2 Objectives and scope of study
The literature part of the study concentrates on collecting the latest information about
PHCs in municipal wastewaters. The experimental part will focus on getting a
laboratory scale sequencing batch reactor (SBR) to operate. The feasibility of the
reactors' operation and sampling procedures are verified by checking mass balances
with the help of a marker compound. A final objective of the study is to estimate a
primary biodegradation rate and biodegradation rate constant of a marker compound in
2. Wastewater treatment
Municipal WWTPs are built to treat wastewater that has been produced under normal
household conditions. Urine, feces and grey water resulting from washing and bathing
are the main sources of municipal wastewater. Industrial wastewaters can also, by
agreement, be led into municipal WWTPs. Industrial wastewaters need in many cases
pretreatment before being led into municipal WWTPs. However, many compounds in
different forms end up in WWTPs. The chemical structure defines how compounds can
be removed. They can be solids and water or fat soluble. It is preferable to separate as
much of the solids as possible before continuing to the next stages of the wastewater
treatment process. The design of WWTPs varies. Biological, chemical and membrane
treatments can all be used separately or combined. (Swedish Environmental Protection
Wastewater can consist of many different compounds in different concentrations
depending on location, time, season and weather conditions. Conventionally wastewater
is seen to consist of suspended solids, biodegradable organics, nutrients (nitrogen and
phosphorus), pathogens, heavy metals, colloidal and dissolved solids, Volatile Organic
Compounds (VOC) and odors. The function of WWTP's is to remove these from
wastewater so that they are not released into the environment. Biological Oxygen
Demand (BOD) and Chemical Oxygen Demand (COD) are used to measure the amount
of organic matter in the wastewater. (Metcalf & Eddy, 2003; Bitton, 2005)
The different treatment steps in a typical WWTP can be divided into preliminary
treatment, primary treatment, secondary treatment, advanced/tertiary treatment and
solids treatment and disposal. The objective of preliminary and primary treatment is to
remove solids by mechanical treatment and by settling or floating. Secondary and
advanced treatments are mostly biological and/or chemical treatment processes that
remove organics, nutrients, pathogens, parasites and toxic compounds from the water.
(Vesilind, 2003; Metcalf & Eddy, 2003; Bitton, 2005) In Figure 1 the treatment process
of the Suomenoja WWTP is illustrated.
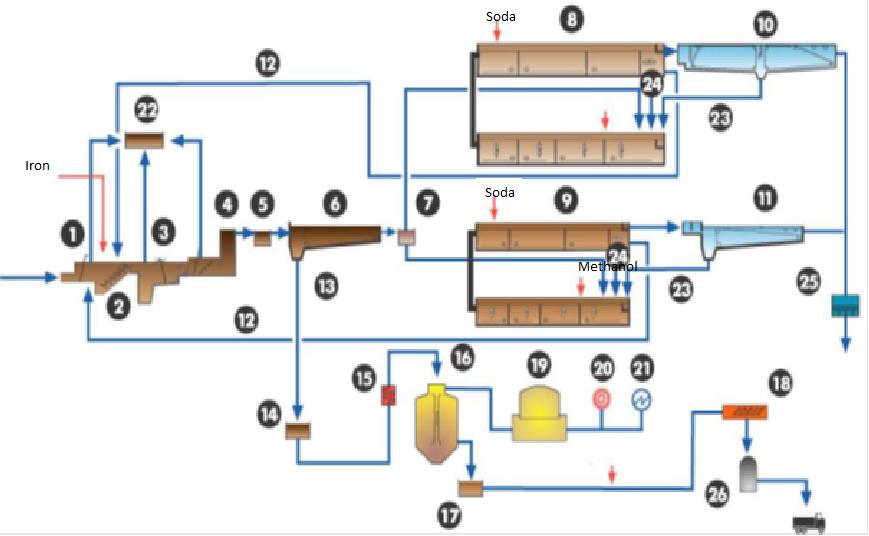
Secondery sedimentation tanks 5…10 21.
Sand - and grit storage
Returned activated sludge
Sludge thickening
Heating of the thickened sludge
Reserved for tertriary treatment
Primary sedimentation
Thickening of digested sludge
Activated sludge chambers 1…4
Centrifugation of sludge
Activated sludge chambers 5…4
Secondery sedimentation tanks 1…4 20.
Figure 1 Operation of Suomenoja WWTP. (Helsinki Region Environmental Services Authority, 2011)
2.1 Biological treatment
In a municipal WWTP the objective of biological treatment is to biodegrade
constituents into acceptable end products, capture solids into biological flocs or biofilm
and to remove nutrients and other organic particulates. Microorganisms in the biological
treatment oxidize organics into more simple constituents. The microorganisms are also
used to remove nitrogen through nitrification and denitrification. Biological treatment
can be divided into aerobic, anaerobic and/or anoxic sections. (Metcalf & Eddy, 2003)
Activated sludge process
The activated sludge process is used in many WWTPs as a secondary treatment. The
principle is that activated sludge oxidizes organic matter into CO2 and H2O, NH4 and
new biomass under aerobic conditions. The biomass forms suspended solids flocs in the
tank, which are removed by settling. Mixed Liquor Suspended Solids (MLSS) is used as
a measure of the solids content of an activated sludge tank. It describes the amount of
organic and mineral suspended solids in the tank, which includes microorganisms.
Nitrification is the process where ammonia is oxidized into nitrite and then nitrite is
oxidized into nitrate. This reaction is needed in a WWTP in order to remove ammonia
due to toxicity and total nitrogen due to eutrophication effects. Because aerobic
autotrophic bacteria, the nitrification bacteria, grow more slowly than heterotrophic
bacteria, nitrification needs a longer Solids Retention Time (SRT). Heterotrophic
bacteria are responsible for organics removal. The nitrification steps are shown in
Equation 1. (Metcalf & Eddy, 2003)
In order to complete the nitrogen removal, which starts with nitrification, denitrification
needs to take place. Denitrification means the reduction of nitrate into nitric oxide,
nitrous oxide and nitrogen gas. The denitrification steps are shown in Equation 2.
(Metcalf & Eddy, 2003)
Phosphorus removal
Phosphorus removal can be achieved both biologically and by chemical addition. The
basic idea is to incorporate phosphorus into suspended solids. This can happen either by
microorganisms or precipitation chemicals such as iron or aluminum salts. The
chemical reactions for precipitation with aluminum and iron can be seen in Equations 3
and 4. (Metcalf & Eddy, 2003)
Biological phosphorus removal can be achieved with many different methods. The
biological phosphorus removal processes include, in the biological suspended growth
process, an aerobic zone followed by an anaerobic zone. The biological removal of
phosphorus can also be achieved by modifications of this basic principle. (Metcalf &
3. Potentially harmful compounds in wastewater
3.1 History
In the 1950s concerns grew over the effects of pollutants in nature. Birds and raptors
were found dead or unable to reproduce. When the animals were tested, high amounts of
the pesticide DDT was found in their bodies. DDT and mercury were used widely in
agriculture as insecticides and as prevention of fungal growth on seeds. High levels of
mercury and DDT were also found in agricultural produce and fish, which led to
growing concerns over their effects on human health. In 1966 a Swedish researcher
found high concentrations of Polychlorinated Biphenyls (PCBs) in the environment.
These compounds have been produced since 1929. In the 1960s and 1970s many of
these toxic pollutants were banned from production and use. These are however still
found in the environment today. (Swedish Environmental Protection Agency, 2008;
Stockholm Convention, 2011)
3.2 Current research on potentially harmful compounds in wastewater
Municipal WWTPs are not generally designed to remove pharmaceuticals or other
PHCs from wastewaters. The use of harmful compounds can be prohibited by
governments and thus eliminating them from wastewaters and preventing them from
ending up in the environment. (Swedish Environmental Protection Agency, 2008) But
to study the fate of these compounds in WWTPs is also important when trying to
control their concentrations in environmental waters. (Urase & Kikuta, 2005)
One of the most important tasks in water research at the moment is to determine what
compounds are harmful and in what concentrations. The problem is that different
organisms can react to a compound in different ways. A concentration of a compound
that is toxic to one organism can be safe to another. Synergy effects of different
mixtures of compounds and their metabolites are also hard to determine or rule out.
Many different things need to be taken into consideration when assessing the risks that
different compounds pose to the environment. Assessments and measurements for
PHCs that should be made are listed below. (Swedish Environmental Protection
Assessment of emission sources and levels Monitoring of levels in environment and organisms, including humans
Exposure assessments Assessments and test on effects on organisms Stability of compound (bioaccumulation and half-life) Determination of harmful levels
In the assessment of the level of threat a compound poses and in what quantities,
Quantitative Structure-Activity Relationship (QSAR) can be used. This model is a way
of calculating the behavior of an unknown compound by comparing its molecular and
physical properties to known compounds. (Swedish Environmental Protection Agency,
2008) The QSAR model has also been used to evaluate the biodegradation of
pharmaceuticals. (Urase & Kikuta, 2005)
In the EU the documentation and knowledge of compounds is growing due to the
REACH-regulation. All chemical products need to be registered and evaluated, and if
they pose any risk their use can be restricted. Therefore it is known what new chemicals
might end up in WWTPs. (Swedish Environmental Protection Agency, 2008)
In 2001 the Stockholm Convention was adopted to influence the problem of Persistent
Organic Pollutants (POPs). The Stockholm convention is a global treaty under the
United Nations Environmental Protection Agency. Originally twelve POPs were
adopted, including DDT and PCBs. In 2009 nine new chemicals were added to the list
in the treaty. Now three more chemicals are considered to be added; chlorinated
naphthalene, hexablorobutadine and pentachlorophenol. Control measures to reduce the
amount of the brominated flame retardant Hexabromocyclododecane (HBCD) and Short
Chain Chlorinated Paraffins (SCCPs) are also being evaluated. (Stockholm Convention,
As POPs are a priority in international agreements, they are now studied more intensely.
POPs are biodegraded very slowly and they bioaccumulate in the environment when
released. They are believed to be toxic, carcinogenic and endocrine disruptive. Because
of their chemical composition they have been shown to accumulate in the fat tissue of
living organisms. They do not volatilize, they have a large molecular weight and are
hydrophobic. (Malmi & Mannio, 2008)
Many of the compounds that have recently started to worry researchers are compounds
with endocrine disrupting tendencies. As early as in the 1930s there have been reports
of natural and synthetic compounds that can mimic endogenous hormones in animals
and disturb the endocrine system. (Liu, et al., 2009) In the 1990s hermaphroditic fish
were found in sewage treatment lagoons. (Johnson, et al., 2005)
Endocrine Disruptive Compounds (EDCs) are among the most harmful of man-made
compounds as they disturb the reproduction of aquatic animals. EDCs have also been
linked to human sperm quality and cancers such as breast-, prostate- and testicular
cancers. EDCs have either estrogenic or androgenic activity and can be harmful even in
very low concentrations. (Liu, et al., 2009)
Many different types of compounds have been observed to have endocrine disruptive
tendencies (pharmaceuticals, personal care products, pesticides, plasticizers, industrial
chemicals etc.). These compounds, that have the ability to mimic or modify endogenous
hormones, are called xenoestrogens. (Combalbert & Hernandez-Raquet, 2010) The
endocrine system is very complex, which can explain why so many different
compounds have shown to disturb it. (Bolong, et al., 2009) Natural and synthetic
hormones are the most potent estrogenic compounds; these are discussed in Chapter 4.2.
3.3 Removal of potentially harmful compounds in wastewater
treatment plants
There are four different things that can happen to organic compounds in a conventional
WWTP. A compound can break down (biodegrade), sorb to sludge, volatilize or end up
in the effluent. Compounds can break down completely (mineralize) or turn to other
compounds. Different operational conditions have been found to influence the
biodegradation of a compound. Some compounds biodegrade better with a long SRT
and the biodegradation is usually faster when a high concentration of the compound is
present. (Swedish Environmental Protection Agency, 2008)
The biological transformation of compounds is dependent on many different things. A
biodegradation rate constant can be used to describe the biological transformation. But
the compounds sorption potential has also a big impact on its fate; the sorption
coefficient kd describes this. Compounds with high kd values need a longer SRT to
accomplish their biodegradation. (Suárez, et al., 2008)
Xenobiotic compounds are particularly persistent to biodegradation and microbes. They
are often halogenated organic compounds that are especially hard for microbes to
degrade. Halogenated organic compounds include halogenated hydrocarbons,
halogenated aromatics, pesticides and PCBs. (Bitton, 2005)
3.3.1 Biodegradation
Biodegradation is biological degradation by either aerobic or anaerobic microorganisms
that leads to the reduction of a parent compound or metabolite. WWTPs have a huge
potential to reduce PHCs and their metabolites via biodegradation. Biodegradation will
mostly occur during secondary treatment in the wastewater treatment process. This is
where the compounds will be subjected to the most activity by microorganisms. The
biodegradability of a compound is largely dependent on its structure. Unbranched
compounds with short side chains are more likely to biodegraded than compounds with
a large number of branched side chains. (Jones, et al., 2005)
Many of the compounds, foreign to the biological systems, that have been introduced to
the environment during the last century are not readily biodegradable. Many factors
contribute to the resistance to biodegrade. Not only the chemical structure but also
environmental factors, toxicity to microbes, unavailability of nitrogen and phosphorus,
low substrate concentration and unavailability of the substrate due to sorption. (Bitton,
Two main mechanism of biodegradation are possible. Co-metabolism takes place when
a compound biodegrades only when other organic compounds are present and are acting
as substrate for the microorganisms. The other mechanism is called catabolic
metabolism and it takes place when the compound in itself can be the only source of
substrate for the microorganisms. (Stasinakis, et al., 2005)
Biodegradation is also highly dependent on the microbial communities. If the
communities are not adapted to the compound they start to select the microbes that can
degrade the compound. This is why a period of acclimation is sometimes needed before
the foreign compound starts to biodegrade. (Bitton, 2005) Even though there have been
research in microorganisms' role in the biodegradation of compounds, it is still unclear
which have the main responsibility in degrading which compound. Therefore it remains
hard to optimize WWTPs for PHCs removal. (Roh, et al., 2009)
Biodegradation of compounds in WWTPs can be described by pseudo first order
reaction illustrated in Equation 5. (Ternes, 2004)
Ci is the concentration of the soluble substances of the compound, kbiol is the kinetic
constant (biodegradation rate constant) and SS is the Suspended Solids concentration.
More about rate equations is described at the end of this chapter.
Biodegradability depends not only on the constants but also the diversity and total share
of the biomass. Typically the biodegradation rate constant in a conventional WWTP
mean the following removal rates: (Ternes, 2004)
kbiol < 0.1 = no removal due to biodegradation
0.1< kbiol < 10 = removal due to biodegradation is dependent on treatment
kbiol > 10 = at least 95% removed by biodegradation
Many studies that have measured the biodegradation of compounds have not studied the
metabolites that may form. By only studying the parent compound and not metabolites
that can be even more harmful than the original compound, important facts may go
undetected. Biodegradation does not explain if a compound mineralizes or transforms to
metabolites. (Fatta-Kassinos, et al., 2011)
Rate equations
As it is not possible on a molecular level to observe and see reactions taking place,
models to describe the reactions must be made. Rate equations can be used to describe
reactions in batch processes. When a reaction cannot happen in both directions, the
reaction is seen as irreversible. For irreversible reactions, the reaction order and a
reaction rate constant can be determined. (Solen & Harb, 2011) To determine the
reaction order and rate, the concentration versus time data need to be analyzed. For this
different methods can be used. (Fogler, 2009)
One way to determine the reaction order and rate equation is to guess (or know it
beforehand) and then integrate the equations that are used to describe the system. When
the reaction order is correct, the plot of the data will be linear. (Fogler, 2009)
In a first order reaction:
C0 is the concentration in the beginning and CA is the concentration at time t. This
means that when ln(C0/CA) is plotted as function of time, the slope is linear. The
reaction rate constant k is the slope of the line. In a second order reaction a plot of 1/CA
against the function of time gives a linear slope. (Fogler, 2009) In a pseudo first order
reaction, that is often used to describe the biodegradation of pharmaceuticals, a reaction
that is initially second order is changed to first order as the other variable, the suspended
solids concentration, can be considered to remain constant. (Fogler, 2009; Ternes, 2004)
When the reaction rate/degradation constant, kbiol, is known, it is possible to calculate
the relative amount degraded in a batch reactor with the Equation 7. Cout is the
concentration at the end. (Ternes, 2004)
The total biological transformation can be calculated with Equation 8.
3.3.2 Sorption
Compounds can either adsorb or absorb to sludge. Adsorption happens when ions bind
or physically adhere to another molecule. For example negatively charged
microorganisms in sludge have the ability to adsorb positively charged compounds. The
chemicals ability to ionize or dissociate to aquatic phase can be characterize by ka (the
dissociation constant). (Suárez, et al., 2008)
Absorption is the reaction when molecules in a fluid enter another bulk phase. The
tendency to absorb is related to the chemicals lipophilicity which can be described with
kow (octanol-water partition coefficient). (Suárez, et al., 2008) For hydrophobic and
positively charged ions, sorption is an important removal mechanism. The log kow value
can be used to describe an organic compound's tendency to sorb to sludge. (Swedish
Environmental Protection Agency, 2008)
Log kow < 2.5, low sorption potential
Log kow 2.5-4, middle sorption potential
Log kow > 4, high sorption potential
The sorption to sludge can also be described with the following equation:
Where Ci, sorbed is the concentration of the compound that is sorbed to the sludge, kd, i is
the sorption coefficient and Ci, soluble is the soluble concentration of the compound.
3.3.3 Volatilization /stripping
Many PHCs are able to evaporate to the atmosphere and stay there for various amounts
of time. A compound's ability to evaporate is determined by its volatility. A volatile
PHC can travel from the original pollution source and end up in another part of the
world. (Swedish Environmental Protection Agency, 2004) Volatilization, or air
stripping, transfers mostly VOC and ammonia from water phase into air phase. (Bitton,
4. Pharmaceuticals and Personal Care Products (PPCP)
4.1 Personal care products
Compounds used in personal care products have lately been frequently detected in water
supplies. Personal care products, including fragrances, hygiene products, cosmetics and
sunscreens may include compounds that have negative environmental effects and act as
EDCs. (Suárez, et al., 2008) Personal care products consist of a lot of different
compounds, including preservatives and antibacterial compounds, and these can cause
negative effects both to the environment and the wastewater treatment process. Also
sunscreen agents and musk's can contain PHCs. (Swedish Environmental Protection
4.1.1 Triclosan
Triclosan is used in many personal care products such as soaps, fabrics, creams and
cleaners. It is used as an antibacterial agent and preservative and is present in most
items that contain antibacterial properties. As the product has been dispersed,
environmental waters have become polluted with the compound. The concern is, that
the presence of triclosan in the environment will help form cross-resistance in
pathogens and that the compound is toxic. Triclosan is believed to be an androgen and
form chlorophenols with free chlorine during drinking water treatment. (Roh, et al.,
2009) The properties of triclosan are presented in Table 1.
Federle et al. (2002) studied the fate of triclosan in a conventional activated sludge
WWTP. They observed that up to 85-95 % of the influent triclosan biodegraded and
1.5-4.5% sorbed to sludge. They concluded that triclosan was biodegradable in both
high and low concentrations in activated sludge. They showed that acclimation of the
microorganisms is crucial to the biodegradation of triclosan. They also concluded that
triclosan did not disrupt the activated sludge processes in the WWTP, not even in high
concentrations. (Federle, et al., 2002)
Paxéus (2004) studied the removal of triclosan in five WWTPs in Europe. All the
WWTPs used primary settling and activated sludge in their treatment. He observed that
triclosan had high removal rates in WWTPs, with an average of 73% and the lowest
removal of 58%. (Paxéus, 2004) It has also been experimentally proven that triclosan
does not biodegrade under anoxic or anaerobic conditions. (Chen, et al., 2011)
In a study made by Roh et al. (2009) the biodegradation of triclosan, bisphenol A and
ibuprofen by ammonia-oxidizing bacteria was studied. They observed that triclosan was
biodegraded by the ammonia-oxidizing bacteria Nitrosomonas europaea. But the test
also indicated that, due to competitive behavior or toxicity effects, the presence of
triclosan reduced nitrate production. (Roh, et al., 2009)
Table 1 Properties of triclosan
Chemical structureMolecular weight
Antibacterial agent
Thomas et al . (2004)
4.1.2 Galaxolide (HHCB)
HHCB is a musk fragrance used in many personal care products. Of a large group of
polycyclic musk's, HHCB is the most commonly used. It is also known by the names
Abbalide, Pearlide and Chromanolide. HHCB is only produced in one factory in the EU
(1000-5000 tons/year) but it is imported from outside the EU. (European Union-Risk
Assesment Report, 2008) The properties of HHCB are presented in Table 2.
HHCB is a growing interest to researchers as it is used in large quantities and has shown
low biodegradability. A conclusive biodegradation rate of HHCB is not known.
(European Union-Risk Assesment Report, 2008) Kupper et al. (2006) found that HHCB
sorbed to the sludge 72% - 86 %; this seems to be the only way of removing the
compound from wastewater. (Kupper, et al., 2006) In the POSEIDON project, the
sorption to sludge was estimated to be about 80%. (Ternes, 2004) There has not been
found any health risks for humans resulting from the use of HHCB. (European Union-
Risk Assesment Report, 2008) HHCB has however shown to be an endocrine disrupter
in fish. (Kupper, et al., 2006)
Table 2 Properties of the musk HHCB
Chemical structureMolecular weight
Suarez et al . (2008)
Suarez et al . (2008)
4.2 Hormones
Studies have detected four main and potent estrogens in wastewater; E1 (Estrone), E2
(Estradiol), E3 and EE2 (17α-Ethinylestradiol). Of these, the three first are natural
hormones and the last one is a synthetic hormone. E1 is a degradation product of E2 and
therefore found in larger concentrations than E2 in the environment. EE2 is used as a
contraceptive and in other hormone therapies. Although synthetic estrogens can be
classified as pharmaceutical compounds, this chapter will only focus on estrogens. More
of other pharmaceuticals can be found in Chapter 4.3. (Racz & Goel, 2010; Johnson, et
al., 2005) Estrogens were concluded to be, in the POSEIDON project, the most
important PPCP to study in WWTPs. (Ternes, 2004) The properties of E1, E2 and EE2
are presented in Table 3.
Kidd et al. (2007) studied the fate of a fish population in a lake when the synthetic
estrogen EE2 was added to a lake to the concentration of 5-6ng/l. They found large
feminization of the fish population and other reproduction disturbing effects. Almost the
entire fish population was extinct within the 7-year experimental period. This shows
that the estrogens are very potent even in very low concentrations. (Kidd, et al., 2007)
Most of the estrogen that is released into nature comes from humans and livestock. The
amount of estrogen that a human excretes depends on a person's sex and age. Pregnancy
increases the excretion of all estrogens. It has been calculated that the total human
population excretes about 29.5t of estrogens a year. This does not include the 720 kg of
synthetic hormones that is released to the environment due to hormonal contraception.
The concentration of estrogens in influent wastewater can be between 1-700ng/l,
depending on country and population structure. (Combalbert & Hernandez-Raquet,
The fate of estrogens in WWTPs is dependent on chemical properties of the hormone,
treatment plant type, processes and properties of the plant, and biological activity.
WWTP parameters that have been shown to influence the fate of the estrogens are SRT,
Hydraulic Retention Time (HRT), temperature, carbon load redox potential and floc
size. Especially SRT has been proven to be an essential parameter. The longer SRT is
linked to nitrification and a hypothesis is that nitrifying bacteria biodegrade estrogens.
(Combalbert & Hernandez-Raquet, 2010) A long SRT also allows more slowly growing
bacteria (including nitrifiers) and a more diverse bacterial community. (Racz & Goel,
2010) It has been experimentally shown that EE2 is degraded in nitrifying activated
sludge even without an adaptation period. Some studies suggest that, while ammonia
and nitrate are present in low concentrations in a WWTP, heterotrophic bacteria are
responsible for estrogen degradation. (Combalbert & Hernandez-Raquet, 2010)
Estrogens can also be subject of sorption after deconjugation. The fate of estrogens in
WWTP is illustrated in Sorbed estrogens easily enter the nature again through
sludge that is disposed in landfills or otherwise in the environment, that is why
biodegradation is preferred as the best way to remove estrogens. (Racz & Goel, 2010)
Although hormones have hydrophobic properties and should therefore sorb into sludge,
it has been shown through mass-balance calculations that only 6-8% of the hormones
sorb to sludge. The primary way to remove estrogens is through biodegradation.
(Combalbert & Hernandez-Raquet, 2010) In activated sludge WWTP it has been
recorded that estrogens are removed from the influent water 18-100%, depending on the
plant and the type of estrogen. (Racz & Goel, 2010) In the POSEIDON project E1 and
E2 were found to biodegrade over 95% in nitrifying activated sludge. EE2 was not
found to be removed at low sludge ages but in nitrifying activated sludge the
biodegradation was over 90%. EE2 was also found to sorb to sludge less than 5%.
(Ternes, 2004) To understand the correlation between sorption and biodegradation of
estrogens in WWTP, more study is needed. (Combalbert & Hernandez-Raquet, 2010)
Two WWTPs were studied in Finland in 1999 by Johnson et al. (2005) for
concentrations and removal rates of E1 and E2. They found up to 45ng/l E1 in
wastewater influent in the Viikinmäki WWTP in Helsinki. The removal rate of E1 was
estimated to be 94%. The treatment of wastewater in Viikinmäki WWTP consisted of
an activated sludge process with an anoxic zone, chemical phosphorus removal and
nitrification. During the sample period in June 1999 the temperature of the effluent
water was 15°C, the organics removal 90-98% and the plant had a SRT of 19 days and a
biological HRT of 8.8 hours. (Johnson, et al., 2005)
Figure 2 Fate of estrogens in WWTPs and environment. (Racz & Goel, 2010)
Table 3 Properties of the estrogens E1, E2 and EE2.
Chemical structureMolecular weight
Suarez et al . (2008) Combalbert et al .
Suarez et al . (2008)
Suarez et al . (2008)
Chemical structureMolecular weight
Suarez et al . (2008) Combalbert et al .
Suarez et al . (2008)
Suarez et al . (2008)
Chemical structureMolecular weight
Razc et al . (2009)
Ternes (2004)Combalbert et al.
Suarez et al . (2008)
4.3 Pharmaceuticals
Pharmaceutical is a generic name for thousands of different products and compounds
that are used for prevention and treatment of diseases. Because of their spread use and
variety, it is hard to detect all of the different pharmaceutical compounds and
understand their fate in WWTPs. It is important to study drugs that due to their wide use
are present in the environment. Some of these may have harmful effects even in small
quantities. Antibiotics in the environment are especially a concern because even when
they are present in only small quantities, they can induce antibiotic resistance in
bacteria. (Jones, et al., 2005)
Pharmaceuticals are a problematic issue and differ from other PHCs in wastewater
treatment. As these cannot be forbidden due to ethical reasons and the use of them is
hard to restrict, other methods to reduce their presence in the environment must be
studied. Pharmaceuticals are also mostly synthetic and made in a way to have a large
biological effect and still be quite persistent. They can also be constructed in a way that
they are not degraded by the micro bacteria in the intestines. Pharmaceuticals have in
many ways the same attributes as pesticides but the big difference is that they are
usually released into the environment after they have been broken down in the body.
(Swedish Environmental Protection Agency, 2008)
Pharmaceutical products are used all over the world to treat humans and animals. There
is little research about how much of these products, their residues or degradation
products come into the sewage treatment plants and through there into water supplies
and nature. Some pharmaceutical residues are believed to get into nature through
pharmaceutical factories and improper disposal of pharmaceutical products. These are
however considered to be small in comparison to the pharmaceutical residues that are
released with the excretion from the users of the remedies. (Jones, et al., 2005)
As pharmaceuticals are made to treat humans and animals, they are constructed in a way
that the body can take them up easily. They are therefore often biologically active,
hydrophilic and persistent so that they do not degrade earlier than wanted. (Urase &
Not all pharmaceutical compounds are biodegradable, but studies have been made and
e.g. paracetamol and ibuprofen, both very common compounds in painkillers and anti-
inflammatory medicine, were found to be largely biodegradable. Pharmaceutical
compounds are also observed to sorb to sludge and if the sludge is not treated, these go
into sludge utilization or disposal and through there into groundwater and environment.
It has been observed that sludge treatment, for example thermal treatments, have
reduced the amount of pharmaceutical compounds in sludge. It is also possible to with
more advanced wastewater treatments reduce the concentrations of these compounds.
The reduction of pharmaceutical compounds is possible in WWTPs today, but the total
removal may be too costly to optimize. The effort to reduce nutrients in wastewaters has
also led to the reduction of pharmaceuticals. (Jones, et al., 2005)
Under normal conditions, there has been no evidence of pharmaceuticals disturbing the
wastewater treatment process, but it can't be excluded that these may in some way have
a negative effect. (Swedish Environmental Protection Agency, 2008) In a study made
by Kraigher et al. (2008) the influence of pharmaceuticals residues on microbial
communities in wastewater treatment was studied. They found that acidic drugs, such as
ibuprofen and diclofenac at levels of 50µg/l reduced diversity and caused shifts in the
structure of microbial communities. They found that these drugs can possibly have an
influence on especially the nitrogen removing bacteria. (Kraigher, et al., 2008)
The consumption of some widely used and studied pharmaceuticals in Finland 2010 can
be found inThe consumption was calculated with Equation 10. (Vieno, 2007)
Table 4 Pharmaceutical consumption in Finland 2010 (Finnish Medicines Agency, 2011)
4.3.1 Ibuprofen
Ibuprofen is a non-steroidal acidic anti-inflammatory drug that is largely used
throughout the world. (Lischman, et al., 2006) Acidic drugs are ionic in neutral pH,
which makes them an interesting compound to study. They do not sorb to sludge
although according to their kow values they should, but this does not take into account
the effect of pH. (Thomas & Foster, 2005)
Ibuprofen is an over-the-counter drug that is used to treat many different illnesses and
symptoms, including fever and headaches. The therapeutic dose of the drug is large (up
to 1200mg/d) and 70-80% of this is excreted unchanged after use. This means that a
large proportion of ibuprofen is getting to WWTPs. (Smook, et al., 2008) The
compound has been detected in Finnish surface water in concentrations up to 65ng/l and
in WWTP influents and effluents 20µg/l and 4µg/l respectively. This is more than in
most other European countries. (Ternes, 2004)
It has been shown that ibuprofen does not volatilize or sorb to the sludge. The main
removal method of ibuprofen is therefore biodegradation. Most of the drug biodegrades
in the aeration tank during secondary treatment in a conventional WWTP with activated
sludge. The total removal of ibuprofen in WWTPs can be up to 99%. (Smook, et al.,
Clara et al. (2005) studied the occurrence and removal of pharmaceuticals in three
different wastewater treatment plants. From one of the three plants, samples were taken
at three different occasions and temperatures. They detected that the biodegradation of
ibuprofen was not affected by the change in temperature between 7-22°C. In this plant,
the SRT was well over 10 days and the food per mass ratio (F/M) was low,
0.02gCOD/gTSS. The removal of ibuprofen was over 90%. However at another plant,
that had an SRT of only 2 days and a high F/M ratio of 1.7gCOD/gTSS, no ibuprofen
removal took place. The plant was design for only organics removal. The influent
concentration of ibuprofen in both WWTPs was around 2400ng/l. (Clara, et al., 2005)
The drug oxidizes to hydroxyl and carboxyl metabolites in WWTPs and this often
contributes to the high removal rates. (Paxéus, 2004) Although the drug has a high
removal rate in activated sludge WWTPs, it is still found in environmental waters due to
the high influent concentration and nowadays low detection limits. (Malmi & Mannio,
2008) The drug has also been found to bioaccumulate in pH values below 5.5. (Det
Norske Veritas, 2006)
Roh et al. (2009) discovered that ibuprofen was not degraded by the ammonia-oxidizing
bacteria Nitrosomonas europaea but it did degrade in nitrifying activated sludge. Some
studies have detected ibuprofen-degrading bacteria but it is not known which strains are
responsible for the biodegradation of ibuprofen in WWTPs. (Roh, et al., 2009)
Table 5 Properties of ibuprofen
a-Methyl-4-[isobutyl] phenylacetic acid
Chemical structureMolecular weight
Anti-infammatory drug
Suarez et al. (2008)
Smook et al. (2008)
kbiol values for ibuprofen
The biodegradation rate constants found for ibuprofen in literature are presented in
The three studies made by Joss et al. (2006), Ternes (2004) and Smook et al.
(2008) studied the biodegradation of ibuprofen in activated sludge. The results were
analyzed as pseudo first order degradation by which the biodegradation rate constant
The study made by Joss et al. (2006) studied the biodegradation of ibuprofen in batch
experiments. Wastewater from WWTPs was spiked to levels of 3µg/l ibuprofen and the
biodegradation of the compound was studied in a batch with MLSS concentration of
3.2g/l. The experiments were made in a laboratory where the temperature was 17 ± 1°C.
The sludge used from a WWTP had a sludge age of 11 ± 1 days and COD concentration
of the influent wastewater was 275mg/l. They obtained the kbiol values of 21-35 l/gSS/d.
(Joss, et al., 2006)
Ternes (2004) studied many different WWTPs in Europe and detected that when the
sludge age was over 5 days, the kbiol values were 23 ± 10 l/gSS/d. The third experiment
by Smook et al. (2008) studied the biodegradation in the aeration tank in a WWTP that
uses conventional activated sludge process for nutrient removal. The HRT in the
aeration tank was 7 hours and the MLSS concentration was on average 2.33g/l. (Smook,
et al., 2008)
4.3.2 Diclofenac
Diclofenac is, as ibuprofen, a non-steroidal acidic anti-inflammatory drug. It has been
noticed that the drug can cause serious effects to animals. A species of birds were
almost extinct in India because of a spill of the compound and it has been shown to
cause liver faults in rainbow trout. (Swedish Environmental Protection Agency, 2008)
In Finnish surface waters the drug is found at lower concentrations than in the rest of
Europe. (Ternes, 2004) Diclofenac has shown poor biodegradation and sorption to
sludge. Biodegradation is still possible under some conditions. A low removal rate of
15-40% has been shown in WWTPs. (Zang, et al., 2008; Ternes, 2004) The poperties of
diclofenac are summarized in Table 6.
Table 6 Properties of diclofenac
2-[(2,6-Dichlorophenyl)amino] benzeneatic
Chemical structureMolecular weight
Anti-inflammatory drug
Suarez et al. (2008)
4.3.3 Paracetamol
Paracetamol, even known as acetaminophen, is an analgesic drug. It is one of the most
commonly used non-steroidal analgesic drugs. Contrary to ibuprofen and diclofenac, it
has a low anti-inflammatory effect. It is metabolized in the liver and can in high doses
be toxic and cause liver failure. (Rang, et al., 1995)
In a study of the removal of pharmaceuticals at a southern English WWTP, it was found
that paracetamol was removed on an average of 92% over a four day sampling period.
The WWTP used activated sludge treatment as their secondary biological treatment
process. They had also nitrification and denitrification zones. The mean temperature at
the time of sampling was 20.6°C and the sludge age 3 days. The concentration found in
influent wastewater was 2-3µg/l. (Jones, et al., 2005) Paracetamol biodegrades fast and
has as high kbiol of 58-80 in activated sludge. (Joss, et al., 2006) The properties of
paracetamol are summarized in Table 7.
In a study made at a WWTP in Spain they found paracetamol at levels of 7.1-11.4µg/l in
the influent. The WWTP received both municipal and industrial wastewaters, which
included pharmaceutical industry. The WWTP used activated sludge as secondary
biological treatment, with both anoxic and aerobic zones. The SRT during the time of
sampling was 10 days and the HRT 11.5 hours. They detected that paracetamol was
removed to up to 99.9 ± 0.1% from the aqueous phase in the WWTP. The study
concluded that due to the fast biodegradation, paracetamol does not sorb to sludge in
significant amounts. (Radjenovic, et al., 2009)
Table 7 Properties of paracetamol
Chemical structureMolecular weight
Jones et al. 2002
Joss et al. 2006
Joss et al. 2006
4.3.4 Carbamazepine
Carbamazepine is used to treat seizures in epilepsy and has been found in many studies
in WWTP effluents and natural waters. Because of its low removal rate in WWTPs, the
compound has been proposed to serve as an anthropogenic marker. Less than 10% of
Carbamazepine is removed in WWTPs and it is often classified as not removable. In
some cases the removal rate has been 30%, but mostly the removal is only 0-10%.
Longer SRT has not been shown to influence the removal of carbamazepine. It neither
biodegrades nor sorbs in any large extent to the sludge in WWTPs and stays therefore in
the aquatic phase and is released with the effluent into nature. (Zang, et al., 2008) The
properties of carbamazepine are summarized in Table 8.
Table 8 Properties of carbamazepine
Chemical structureMolecular weight
Anti-epileptic agent
Suarez et al. (2008)
Suarez et al . (2008)
4.3.5 Antibiotics
Antibiotics are drugs that are used to treat and prevent microbial infections both in
humans and animals. A large amount of antibiotics that are used, are excreted
unchanged and transferred to WWTPs. Antibiotics are also used in breeding of livestock
animals, in feed additives and in the poultry industry. The biggest concern with letting
antibiotics into the environment, and the effects on human health, is the risk of
antibiotic resistant bacteria developing. (Lindberg, et al., 2005; Kim & Aga, 2007) A
high number of antibiotic resistant bacteria can be found in wastewaters. Here different
bacteria from many different individuals have an opportunity to react with each other
and this has potential genetic consequences. Studies have shown that antibiotic resistant
bacteria favor the conditions at a WWTP and this may lead to the transfer of the
antibiotic resistant gene to other bacteria. The conditions at WWTPs have been shown
to lead to a greater likelihood of transfer of the gene. (Kim & Aga, 2007; Fatta-
Kassinos, et al., 2011)
Fluoroquinolones is a group of antibiotics that are largely used in Europe and especially
Scandinavia. They have been observed to mostly sorb to sludge and have a very low to
none biodegradability in conventional WWTPs. (Lindberg, et al., 2005; Vieno, et al.,
2007) In a research made by Vieno et al. (2006) the fluoroquinolones ciprofloxacin,
norfloxacin and ofloxacin were found in both effluents and influents at WWTPs, surface
waters and in ground- and tap water in Finland. They also detected that over 80% of
these fluoroquinolones were removed in WWTPs in Finland. They did not research how
the antibiotics were removed from the wastewater. (Vieno, et al., 2007) Similar removal
rates have also been observed by Lindberg et al. (2005) in Swedish WWTPs. The
properties of the fluoroquinolones ciprofloxacin, norfloxacin and ofloxacin are
summarized in Table 9.
Table 9 Properties of ciprofloxacin, norfloxacin and ofloxacin
Chemical structureMolecular weight
Vieno et al. (2006)
Vieno et al. (2006)
Chemical structureMolecular weight
Vieno et al. (2006)
Vieno et al. (2006)
de]-1, 4-benzoxazine-6-carboxylic acid
Chemical structureMolecular weight
Vieno et al . (2006)
4.3.6 β-Blockers
β-blockers are drugs used to treat high blood pressure and in treatment after heart
attacks to prevent new attacks. The removal of these drugs in WWTP have become
increasingly interesting, as they have been found in natural waters and ecotoxicological
tests have shown them to be toxic to algae and aquatic animals. β-blockers are
positively charged at pH values of around 7 and weak bases. (Maurer, et al., 2007)
Metoprolol
Metoprolol is the most used β-blocker in Finland and it has been found in natural
waters, lakes and WWTPs. (Vieno, et al., 2007) In experiments made by Maurer et al.
(2007) it was shown that metoprolol does not sorb to sludge in significant amounts and
that the main way of removal in WWTP is by biodegradation. The biodegradation rate
constant, kbiol, of metoprolol was calculated to be 0.58l/gSS/d. The compound's
biodegradation is between 25-70% of the influent concentrations. (Maurer, et al., 2007)
Wick et al. (2009) detected that the biodegradation of metoprolol takes place in the
nitrification tank in a conventional WWTP. The properties of metoprolol are
summarized in Table 10.
Table 10 Properties of metoprolol
quinolinecarboxylic acid
Chemical structureMolecular weight
Vieno et al. (2006)
Maurer et al. (2007)
4.3.7 X-ray contrast media
X-ray contrast media are used in radiological examinations. They are a major part of the
pharmaceuticals that leave hospitals with wastewater to WWTPs. As they are only used
in human medicine, WWTPs are most likely the only source of X-ray contrast media
contamination in the environment. (Ternes & Hirsch, 2000)
Iopromide
Iopromide is an X-ray contrast that is used when examining blood vessels and organs.
The compound is very stable and almost all of it is excreted out of the human body
unchanged within a day. (Batt, et al., 2006) Hospital wastewater effluent is the major
source for iopromide to WWTPs as the compound is used only at hospitals for
diagnostic purposes. (Fatta-Kassinos, et al., 2011)
In Germany in a study made at a WWTP close to Frankfurt am Main, iopromide was
found in wastewater influent and effluent at concentrations of 7.5±1.5µg/l and
8.1±1.6µg/l respectively. No removal could be shown of the compound in the
wastewater treatment process. (Ternes & Hirsch, 2000) Iopromide is believed to go
through the WWTP largely unchanged. (Batt, et al., 2006) It has shown some capability
to biodegrade but the results are contradictory and need further research. (Ternes, 2004)
The properties of iopromide are illustrated in Table 11.
Table 11 Properties of iopromide
Chemical structureMolecular weight
Joss et al. (2006)
5. Emerging industrial potentially harmful compounds
5.1 Nonylphenol (NP)
Nonylphenol (NP) is a xenobiotic compound found to have many harmful effects. Most
NP has its origin as a degradation product from Nonylphenol Ethoxylates (NPE) that are
used as industrial surfactants, detergents, cleaners and degreasing agents. NP found in
the environment is a strong sign of human activity, such as wastewater treatment,
nearby. NP is a hydrophobic compound that adsorbs to sludge solids and does hardly
degrade. It is possible for the compound to be degraded by microbial activity but the
biodegradation is strongly dependent on oxygen and bioavailability of NP. (Soares, et
al., 2008)
NP has been found to be toxic and bioaccumulate in fish. It has also been found to
disturb the hormonal system in many animals and organisms and is classified as an
EDC. (Swedish Environmental Protection Agency, 2004) The use of NPE is restricted
in the EU and it is listed as a priority substance. NPEs have been changed to other, not
as harmful, surfactants. Regulatory steps for reducing the amount of NP in the
environment have also taken place in some parts of the world, such as the USA, but in
many countries the production and use of NPE is still taking place. (Soares, et al., 2008)
The properties of NP are summarized in Table 12.
In the E-PRTR study, a study of harmful compounds in Finnish wastewaters, the
concentration of NP was measured at 26 different WWTPs. The average concentrations
of NP/NPE in influent wastewater was 181.91µg/l and in effluents <1.12µg/l. (VVY,
According to the Simple Treat model for WWTPs, 24% of NP biodegrade in
wastewater treatment. (Malmi & Mannio, 2008) In other studies the main removal of
NP has been detected to be through sorption to sludge. (Soares, et al., 2008)
Table 12 Properties of nonylphenol
Chemical structureMolecular weight
Metobolite of alkyl phenols
Soares et al. (2008)
5.2 Organotin (OT) compounds
Organotin (OT) compounds are a group of over 800 different compounds, almost all of
them man-made. (VVY, 2008) OT compounds are used in many applications including
biocides, agrochemicals, antifouling paints, UV and thermal stabilizers and in the
production of foams and silicone. There are many different OT compounds including
Tributyltin (TBT), Dibutyltin (DBT) and Monobutyltin (MBT). TBT is toxic and the
levels of TBT found in marine and freshwater environments are a growing concern.
(Stasinakis, et al., 2005)
In the EU, the use of OT antifouling paints on vessels is forbidden and after 2008 ships
entering EU ports were forbidden to use these paints. Also biocides that use TBT are no
longer on the market in the EU. The use of other OT compounds, in consumer products
and as wood preservatives, is a rising concern in the EU. (RPA, 2005)
OT compounds slowly biodegrade in sediment, especially in cold conditions. Both
biological and chemical degradation decomposes OT compounds. The concentrations of
OT compounds and especially TBT in 10 WWTPs were studied in the E-PRTR study in
Finland. The average of OT compound concentration in influent water was 0.084µg/l
and in effluent water 0.009µg/l. TBT was found in influent wastewater on an average of
0.005µg/l and in effluent < 0,003µg/l. (VVY, 2008)
Stasinakis et al. (2005) studied the biodegradation of OT compounds in a SBR with
both acclimatized and non-acclimatized activated sludge. They found that TBT first
accumulated to the sludge but started to biodegrade during the experiment. This same
happened to DBT and MBT but not in the same extent. When using non-acclimatized
activated sludge the biodegradation was much lower. They also tested the
biodegradation in the presence and without supplemental substrate. They found that OT
compounds can be used as a single carbon source in the activated sludge process. The
presence of supplemental substrate only enhanced the biodegradation of TBT, but did
not affect the biodegradation of the other tested OT compounds. When using
acclimatized activated sludge TBT, DBT and MBT biodegraded 99.7%, 90.4% and
49.7% respectively. (Stasinakis, et al., 2005)
5.3 Perfluorinated compounds
Perfluorinated Alkyl Compounds (PFAS) are a large chemical group of emerging
pollutants with different toxicity and behaviors. They are found in the environment all
over the world and are mostly very stable compounds. PFAS chemical structure is made
of a hydrophilic head group with a completely fluorinated alkyl chain. Because of the
fluorinated structure they are not biodegraded in the WWTPs. PFAS are used in
cleaning supplies, firefighting foams and in the textile industry. (Finnish Ministry of the
Environment, 2005; Sáez , et al., 2008)
The most common PFAS in WWTPs are Perfluorosulfonate (PFOS) and
Perfluorooctanoic Acid (PFOA). PFAS have been found in Finnish seawater and rain up
to 37ng/l. Concentrations of 2.5ng/g solids has been found in WWTP sludge. (Korkki,
2006) In a study that measured concentrations of PFAS in six different WWTPs in New
York State, concentrations of up to 68ng/l of PFOS and 700ng/l PFOA were found in
effluent waters. (Sinclair & Kannan, 2006)
PFOS and PFOA have been found to accumulate in the liver and they are carcinogenic.
They have a lot in common with POPs. They have been found in fish, seals and even in
humans. Especially PFOS is persistent, toxic and bioaccumulates in the environment.
(Korkki, 2006) The use of PFOS is forbidden (with some exceptions) in Finland since
2008 and its use in firefighting foams was stopped in 2011. (SYKE, 2008) Firefighting
foams were the biggest source of PFOS in Finland in 2004. (Korkki, 2006)
PFOS does not biodegrade and most of it will leave a WWTP in the effluent. According
to the Simple Treat model, about 28% of PFOS will sorb to sludge. (Malmi & Mannio,
2008; Finnish Ministry of the Environment, 2005; Sáez,et al., 2008) The only way to
remove PFOS is by burning in high heat. The exact temperature for this to take place
has however not been determined. (Korkki, 2006)
5.4 Bisphenol A (BPA)
Bisphenol A (BPA) is a compound largely used in the manufacturing of resins and
plastics. It is mostly used to manufacture polycarbonate. Polycarbonate is a rigid plastic
that is used in different types of food containers. BPA is also used as a coating agent in
other food and beverage cans. This is an important source of BPA to humans, as
residues of the compound can migrate from the containers. In January 2011 the
European Food Safety Authority (EFSA) banned the production and import of baby
bottles that contain BPA. (European Food Safety Authority, 2011)
BPA is an EDC and has been found to be toxic to aquatic animals in concentrations of
1-10µg/l. (Roh, et al., 2009) BPA has been found to biodegrade in lab-scale
experiments with aerobic activated sludge up to 72-99%. (Liu, et al., 2009) In the study
made by Roh et al. (2009) it was detected that BPA is biodegraded by the ammonia-
oxidizing bacteria N. europeae. Also other bacteria found in wastewater treatment
plants have been found to biodegrade BPA. (Roh, et al., 2009) The properties of BPA
are summarized in Table 13.
Table 13 Properties of BPA
Bisphenol A
Chemical structureMolecular weight
Residual and intermidiate in production of
flame retardants etc.
Urase et al . (2005)
5.5 Polycyclic Aromatic Hydrocarbons (PAHs)
Polycyclic Aromatic Hydrocarbons (PAHs) are formed during incomplete combustion
of organic material. PAHs form during all sorts of combustion from forest fires to diesel
engines. PAHs can also be found in crude oil and in wood preservatives. They form a
group of many different compounds and most of them are carcinogenic and can cause
genetic damage. They are also mostly persistent and in some cases bioaccumulating.
PAHs are dispersed everywhere in the world and the environment. (Swedish
Environmental Protection Agency, 2004) PAHs include toluene, benzene, xylene,
naphthalene etc. (Bitton, 2005)
PAHs are easily evaporated and dissolve poorly to water. In WWTPs they biodegrade
slowly under aerobic conditions and partly sorb to sludge. In the E-PRTR study about
harmful compounds in Finnish WWTPs, PAHs were found in influent and effluent
wastewater on an average of <0.087µg/l and <0.026µg/l respectively. The reduction of
PAHs in the plants varied from 50-98% with a median of 90%. (VVY, 2008)
5.6 Phthalates
Phthalates are used as plasticizers mainly in PVC production but also in production of
paints, PPCPs, glues and lubricants. (Roslev, et al., 2007) Due to their large use,
phthalates can be found in the environment all over the world. They have been found to
be toxic to algae and in large quantities to be endocrine disruptive. It has been noticed
however, that they metabolize rapidly in vertebrates, so they should not be harmful.
(Swedish Environmental Protection Agency, 2004) As the phthalates are most often not
bound to a product, the compounds can escape to the environment in any phase of a
products lifecycle. They have also been shown to bioaccumulate in the environment.
(Roslev, et al., 2007)
Most discussed and tested phthalates are Dibutyl Phthalate (DBP), Butylbenzyl
Phthalate (BBP) and (di-8a-ethylhexyl) Phthalate (DEHP). (Roslev, et al., 2007;
Swedish Environmental Protection Agency, 2004; Malmi & Mannio, 2008; Finnish
Ministry of the Environment, 2005) According to the Simple Treat-model for WWTPs,
DEHP, DBP and BBP biodegrade 15%, 58% and 57% respectively. (Malmi & Mannio,
2008; Finnish Ministry of the Environment, 2005) In experiments made by Roslev, et
al. (2007) they observed a total degradation (biodegradation in activated sludge process
and degradation in sludge digestion) of 81%, 91% and 90% for DEHP, DBP and BBP.
(Roslev, et al., 2007)
Marttinen et al. (2003) studied the removal of DEHP at Suomenoja WWTP and
detected a biodegradation of 29% in the activated sludge process and a 32%
biodegradation in sludge digestion. The temperature at the treatment plant during the
sampling period was 18.5°C, the mechanical HRT was 4 hours, the biological HRT 20
hours and the aerobic nitrification sludge age was 7 days while the total sludge age was
14 days. (Marttinen, et al., 2003)
The most commonly used phthalates are biodegradable but also sorb to sludge under
some WWTP conditions. They are also found in effluents of WWTPs. (Finnish Ministry
of the Environment, 2005) Phthalates that have longer chains are more likely to sorb to
sludge than biodegrade. (Swedish Environmental Protection Agency, 2008)
5.7 Brominated flame retardants
Brominated flame retardants are used in electronic equipment, plastics, rubbers, textiles
and fibers. Their use has increased in recent decades due to computerization and stricter
fire and safety standards. These compounds have been found in most places in the
environment. Some of them have been found to bioaccumulate, be carcinogenic and
neurotoxic. However more research is needed to understand the effects of these
compounds. As there are no other as effective options to use as flame retardants, the
amount of these are expected to increase in the environment. (Swedish Environmental
Protection Agency, 2004; Koskinen, et al., 2005)
The first brominated flame retardants to be used were Polybrominated Biphenyls
(PBBs). The use of these has decreased as they were found to be very toxic when an
accident with the chemical killed livestock. After that the use of Polybrominated
Diphenyl Ethers (PBDEs) started and they were also found to bioaccumulate and have
harmful effects. (Swedish Environmental Protection Agency, 2004) The PBDEs are
mostly banned by the Stockholm Convention. Today the most used brominated flame
retardants are Tetrabromobisphenol A (TBBP-A) and HBCD. (Finnish Ministry of the
Environment, 2010) According to Simple Treat-model for WWTPs, TBBP-A does not
biodegrade but mostly sorbs to sludge (80-90%). (Malmi & Mannio, 2008)
5.8 Chlorinated paraffins
Chlorinated paraffins are a group of compounds that are used for different industrial
applications throughout the world. They are used as components in lubricants, coolants
in metal cutting, plasticizers, flame retardants in plastics, rubber, paints, coatings,
adhesives and sealants. (United States Environmental Protection Agency, 2009)
Chlorinated paraffins are divided into three groups after their carbon chain length;
Short-Chain Chlorinated Paraffins (SCCPs), Medium-Chain Chlorinated Paraffins
(MCCPs) and Long-Chain Chlorinated Paraffins (LCCPs). Of these, SCCPs are
considered to be the most harmful and in the EU regulatory measures to lessen their
production and use has been made. (United States Environmental Protection Agency,
2009) SCCPs are found in biota, water and many other places in the environment. They
are present even in remote places like the arctic. (Stockholm Convention, 2009)
SCCPs have been found to behave like POPs. They bioaccumulate and sensitive aquatic
animals can be effected even at relatively low concentrations (<5µg/l). There is no
conclusive evidence that SCCPs pose any risks to humans, although some research
suggests that they may be carcinogenic. (Stockholm Convention, 2009)
The fate of SCCPs and other chlorinated paraffins in WWTPs have been scarcely
studied. Most of SCCPs that come in the influent wastewater seem to end up in the
sludge. A fraction of the influent SCCPs ends up in the effluent wastewater. WWTPs
are however a big source of SCCPs that ends up in the environment. (Zeng, et al., 2011)
SCCPs have been found in influent and effluent wastewater in Japan 220-360ng/l and
16-35ng/l respectively. In Canada concentrations of 59-448ng/l have been found in
effluent wastewater from WWTPs. The higher concentrations are found in
industrialized areas. (Stockholm Convention, 2009)
6. New research areas
6.1 Sucralose
The artificial sweetener sucralose has been used since 1999, when the United States
Federal Drug Administration permitted it for use. Today it is used in more than 80
countries and in thousands of different products. Sucralose has been shown to be
excreted almost unchanged from the human body. Although it was proven that the
sweetener is safe to use, it has now been shown to affect the gut fauna in rats. This can
mean that it has a disturbing effect on microbes. (Torres, et al., 2011)
The only way of removing sucralose in a WWTP is believed to be via biodegradation.
Due to its hydrophilicity it does not sorb to sludge. Tests have also shown that sucralose
does not biodegrade in large amounts in WWTPs and it has already been found in
significant amounts in surface waters in countries it is used. (Torres, et al., 2011) The
compound has been proposed to be used as an anthropogenic marker due to its
sustainability and because it is only produced synthetically. (Soh, et al., 2011) Sucralose
has been detected in Swedish sewage treatment plant influents 3.5-7.9µg/l and in
Finnish rivers in concentrations up to 0.1µg/l. (Loos, et al., 2009; Brorström-Lundén, et
al., 2008) The properties of sucralose are collected in Table 14.
In a Swedish research of 25 WWTPs where sludge and influent and effluent wastewater
was sampled, it was found that sucralose was removed under 10% in the wastewater
treatment process. The compound was also found in some samples in small amounts in
sludge. It was however concluded that sucralose does not sorb, in significant amounts,
to sludge. (Brorström-Lundén, et al., 2008)
Table 14 Properties of sucralose
Chemical structureMolecular weight
Artificial sweetener
Brorström et al .
6.2 Nanoparticles
Nanoparticles are compounds of the size of 1-100nm. (Finnish Ministry of
Environment, 2010) Nanoparticles are a growing research area for both industry and
scientists. They have a large potential for different applications but the toxicological
effects have not been studied. It is estimated that the use of engineered nanoparticles
will increase in the future. (Kummerer, et al., 2011)
Nanoparticles, due to their small size, can exhibit properties that the material does not
have in bigger size. Also manipulation of a material at nano level makes different
scenarios and applications possible. Nanotechnology can and is used in medication,
engineering etc. to get more precise properties. It has however not been studied what
these engineered properties can have for effects on humans or nature when they are let
into the environment. (Albrecht, et al., 2006)
The most used engineered nanoparticles are nanosilver and TiO2. Nanosilver has
antifungal, antimicrobial and antiviral properties. (Mueller & Nowack, 2008) As
antibiotics resistance is a growing risk due to the extensive use of antibiotics and
antimicrobial compounds, researchers try to find other alternatives to these. As
nanosilver has shown antimicrobial properties its use is growing. (Khan, et al., 2011)
TiO2 is used in paints, coatings and is very common in sunscreens. As TiO2 exhibits
self-cleaning and antifouling properties, these make it a desired compound. (Mueller &
WWTPs have an important role in preventing large amounts of nanoparticles from
being released into nature. It has been proven that large amounts of nanosilver are being
released into WWTPs when washing socks that contain nanosilver. However research
on the fate of nanoparticles in WWTPs is lacking. Preliminary research implicates that
nanosilver is most likely to sorb to sludge. (Kim, et al., 2010)
6.3 Advanced treatments
Conventional WWTPs were not designed to remove the emerging contaminants that
lately have raised concerns. This is why other, more advanced treatments, are being
studied to determine if these could prevent PHCs from entering the environment.
(Bolong, et al., 2009) Some possible advanced treatments are listed below.
Membrane Bioreactors (MBR) Activated carbon Oxidation with ozone Ultra-Violet (UV) photolysis Ion-exchange
All the above-mentioned treatments have been tested on different types of emerging
pollutants and can be more effective than traditional methods. The results are however
largely dependent on the compound and its chemical structure. Some, like ion-exchange
and UV photolysis have shown to be effective but insufficient. (Bolong, et al., 2009)
A pilot scale ozonation plant was added to an Austrian WWTP when it was found that it
did not remove significant amounts of micropollutants. The plant had upgraded to
include nitrogen removal when it was found that this increased the removal of
micropollutants, but not significantly enough. The use of ozonation enhanced the
removal of diclofenac and carbamazepine. (Shaar, et al., 2010)
According to Jones et al. (2007) the implementing of advanced treatments for the
removal of PHCs, like pharmaceuticals and EDCs, may not be beneficial. Although the
removal rate may be very good with advanced treatments, the increase in CO2 emissions
and high costs may lead to more negative effects than the PHCs would have caused. As
traditional treatment methods may not be as effective, they can still remove large
concentrations of PHCs, if designed correctly. (Jones, et al., 2007)
6.4 The future of pharmaceuticals
One way of eliminating the possible negative effects that pharmaceuticals have on the
environment, is to develop environmentally safer and more sustainable pharmaceuticals
i.e. "green" pharmaceuticals. In Sweden a development toward more sustainable use of
pharmaceuticals has started with a new way of categorization. Regulatory agencies
together with the chemical industry have developed a labeling system that describes the
Persistence, Bioaccumulation and Toxicity (PBT) of a pharmaceutical. The scale goes
from 0-9 and the higher the score, the more dangerous the compound is for the
environment. This way doctors and consumers can decide to use a drug that has less
environmentally hazardous effects than another but with the same physiological effects.
(Fatta-Kassinos, et al., 2011)
A study made by Larsson et al. (2007) sampled the effluents of a WWTP in Patancheru
in India that received the wastewater from 90 bulk drug manufacturers. In this study
high levels of pharmaceuticals, especially of the antibiotics (fluoroquinolones), were
found. Ciprofloxacin was found at concentrations of 28000-31000µg/l. This
concentration is higher than the largest therapeutic levels in human plasma. Before it
was thought that the municipal WWTPs and improper disposal of drugs were the largest
sources of pharmaceuticals in the environment. But very little research has been made
of the effluents from drug manufacturers. The drugs manufactured in this area of India
are distributed globally and used in the products of other pharmaceutical companies.
(Larsson, et al., 2007)
7. Sequencing batch reactor
7.1 History
The Sequencing Batch Reactor (SBR), a semi-continuous wastewater treatment process,
was developed in the 1920s in the United States and became more popular in the 1990s.
It is preferred in places where there is not much room for treatment facilities or square
footage is expensive. SBRs are also effective when the flow rates vary largely with
time. Especially milk farms and other types of farms that need their own wastewater
treatment facilities prefer SBR as they do not take up much space, it is easy to decide
when to have it running and control the flows. (Wilderer, et al., 2001) Other places
where SBR technology is and could be used is in treating landfill leachate and
wastewaters from breweries, tanneries, wineries etc. (Mace & Mata-Alvarez, 2002)
7.2 The treatment process
The treatment processes in SBRs are all taking place in one single reactor and the
process bases on a fill-and-draw principle. This is why SBRs are space saving. SBRs
are based on the activated sludge process. The wastewater treatment in SBRs is
organized in timed and controlled sequences. Equalization, biological treatment and
clarification all happen timed one after other. This differs from a continuous activated
sludge process where the different treatment processes takes place in separate tanks and
the wastewater continuously flows through the system. (United States Environmental
Protection Agency, 1999)
The SBR treatment process consists of five stages; fill, react, settle, draw and idle. The
treatment steps can be seen inBefore the treatment in the batch reactor, the
influent wastewater is usually led through screens and grit removal to remove the
biggest constituents as in a conventional municipal wastewater treatment plant. (United
States Environmental Protection Agency, 1999)
Figure 3 The five steps in a SBR.
During the fill stage the reactor is filled with influent wastewater. The batch is already
half-full with sludge and water from the previous cycles. The influent wastewater is a
source of substrate for the activated sludge and sets the biological reaction going. The
fill stage of the process can be created in different ways depending on if aeration and/or
mixing are used. Static fill can also be chosen, which means that no aeration or mixing
is taking place. The decision if aeration is used in the fill stage, depends on if anaerobic
or aerobic conditions are preferred in the beginning of the process. (New England
Interstate Water Pollution Control Commission, 2005)
During the react stage, aeration makes removal of organics and nitrification possible
and anoxic conditions without aeration (with mixing) enable denitrification and thus
leading to total nitrogen removal. Alternating anaerobic and aerobic conditions also lead
to biological phosphorus removal by polyphosphate organisms. During the third step,
both aeration and mixing is turned off to let the sludge settle and clear the water. There
are no other currents interfering with this process as there is no effluent or influent flow
to the SBR. (United States Environmental Protection Agency, 1999)
In the draw stage effluent is taken away from the tank. Different types of decanters can
be used for this purpose. The amount of effluent water to be removed varies depending
on the design and scale of the SBR. The effluent water in the decant stage should be the
same volume as the influent in the fill stage. One recommendation is that no more than
a third of the operating volume should be emptied in the draw stage in a SBR process.
The final, idle stage is between the draw and fill stages. The length of this stage depends
on the chosen operational strategies. (New England Interstate Water Pollution Control
Commission, 2005)
Sludge wasting is also an important step in the process. This can occur at any stage of
the cycle but it has been recommended that the wasting should take place during the
reaction stage. The amount of the sludge wasted, depends on the selected SRT. Because
the aeration and settling stages both occur in the same tank, there is no need for
Returned Activated Sludge (RAS) to be fed to the system. (Metcalf & Eddy, 2003)
7.3 The design
When building an SBR plant for industrial- or municipal wastewater, pilot studies are
recommended. By evaluating the treatability of the influent wastewater and optimizing
the operation beforehand, possible cost savings can be made during the actual building
of the plant. (Wilderer, et al., 2001)
In an SBR process it can be necessary to add alkalinity as nitrification uses up the
naturally existing alkalinity in the water. There are several options of chemicals to
choose from. Sodium bicarbonate, sodium carbonate and calcium hydroxide are all
examples of chemicals that can be used to add alkalinity to the SBR process. The choice
of chemical depends on the process and the preferences of the operation personnel.
(New England Interstate Water Pollution Control Commission, 2005)
7.4 Advantages and disadvantages
SBRs can be cost effective as all the process stages are in one single reactor. This means
that no big clarifiers or other tanks need to be built. SBRs are also very flexible and
easier to control than regular activated sludge processes. A disadvantage is however that
much maintenance, good timing and control systems and knowledge of the process is
needed. The potential of plugging in devices and pipes is also a risk in SBRs. (United
States Environmental Protection Agency, 1999)
8. Description of methods used in analyses of potentially harmful
compounds
8.1 Analyzing PHCs in wastewater
To analyze small concentrations of pharmaceuticals and other PHCs in water pose a
special challenge due to the low concentrations present. Recently different methods
have been developed to make this possible. Solid Phase Extraction (SPE) with Liquid
Chromatography (LC) and Mass Spectrometry (MS) has shown to be an effective
method for sample preparation and analysis of pharmaceuticals in water matrixes. (Batt,
et al., 2008; Farré, et al., 2007)
8.1.1 Solid Phase Extraction (SPE)
Many of the PHCs are dissolved into lipids. To be able to analyze these, methods to
extract the compounds need to be made. SPE is a method where solids and liquids are
partitioned. SPE can be done manually, but automatic SPE by analyzing equipment has
been developed. (Beruetta, et al., 1995)
The reasons for needing SPE are many. SPE can be used when wanting to remove
interfering compounds from samples, to get higher concentrations of e.g. organic
pollutants (pre-concentration), to fraction different groups of compounds in a sample, to
store analytes that are unstable in liquids and to derivatize reactions between reactive
groups of the analytes. (Beruetta, et al., 1995)
The SPE process includes five steps. First, the sorbent is activated by letting it pass
through a solvent the function of which is to condition the solids surface. Then the
solvent is removed by liquid with similar composition as the sample matrix. The third
step is the application of sample, or the sorption or retention step. Here the sorbent will
retain the analytes. After this, the interfering compounds that were retained are removed
with a sorbent that does not remove the analytes. The last step is the elution step where
the analytes are eluted from the adsorbent using a solvent. (Beruetta, et al., 1995)
8.1.2 Liquid Chromatography (LC)
LC is used to fraction samples with different properties. The principle of this method is
that different compounds travel through liquids at different speeds and this way,
different compounds can be separated from each other. The most commonly used
separation technique when analyzing pharmaceuticals in water samples is High
Performance Liquid Chromatography (HPLC). HPLC uses pressure to accelerate the
particles. (Batt, et al., 2008)
Ultra Performance Liquid Chromatography (UPLC) is a new technique and improved
version used instead of HPLC. In UPLC higher pressure is used together with small
particle sized columns. The advantage of UPLC is that it can be used in batch analysis.
It has also shown to have better sensitivity, resolution, reduces analysis time and the
need of solvents. (Batt, et al., 2008)
8.1.3 Mass Spectrometry (M/S)
M/S is used to detect compounds, such as pharmaceuticals. Tandem mass spectrometry
MS/MS is the most frequently used detection system. Mass spectrometry is used after
sample preparation such as SPE and HPLC and can assess the mass of a compound
detected in LC columns. LC-MS is has been an important method for detecting new
pharmaceuticals in the environment. (Batt, et al., 2008) A problem with LC-MS is the
unknown effects of matrixes and their disturbance to the process. A way of
compensating the loss is using internal standards in analysis. (Farré, et al., 2007)
The principle of MS is to bombard the columns with electrons and ionize them. This
way they break up into ionized fragments and the mass spectrometer can detect the mass
of different compounds and identify them. (Swedish Environmental Protection Agency,
Experimental part
9. Materials and methods
9.1 Objective of the SBR plant study
The results from the master's thesis made by Anna Lehtonen (2011) showed the
difficulty of laboratory practices when measuring compounds in low concentrations
(µg/l). In the thesis BPA and HHCB were added to a bioreactor to then find out how the
added compound changed the microbial communities. Because only a fraction of the
added BPA and HHCB concentration could be traced in the influent water, no definite
conclusions could be made in the thesis. However, this finding emphasizes the
importance of planning of experiments. (Lehtonen, 2011)
The objective of the SBR study in this thesis is to trace the added marker compound so
that laboratory practices can be verified. When this has been done, a preliminary
estimation about the biodegradation rate and biodegradation rate constant of the chosen
marker compound can be made. Also one of the main objectives of the thesis is to
study the SBR plants operation and to optimize its parameters and functionality.
9.2 Marker compound
The criteria used in the selection of the marker compound were chosen basis on the
literary review presented in the literature part in this thesis and the experiences in the
master's thesis by Anna Lehtonen (2011). Ibuprofen was chosen because it met the
criteria which made reliable measurements possible. The criteria used are described
A previously studied compound Biodegradable, though not at a too fast rate Does not sorb to sludge in significant amounts Largely used in Finland Have potentially harmful effects in nature
Ibuprofen is a very common drug in Finland. It is used here more than in most other
European countries. (Ternes, 2004) As described in Chapter 4.3.1, ibuprofen is
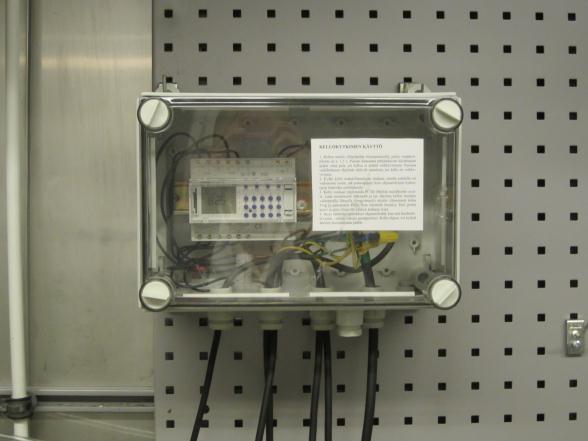
biodegradable in activated sludge and does not sorb in significant amounts to sludge. It
is also a largely studied compound with biodegradation rate constants comparable to the
ones obtained in this study.
9.3 The SBR laboratory plant
The experimental part of the thesis was made in a laboratory scale SBR pilot with four
reactors where the biodegradation of ibuprofen was studied. The SBR was operating
2.11.2011-20.2.2012. The SBR arrangements are illustrated in Figure 7 and pictures in
Figure 8. The parameters for the operation of the SBR were chosen after a literature
study and to best represent the conditions found in Finnish WWTPs. At the beginning of
the experiment, to get the reactors started, sludge from Suomenoja WWTP was added.
After this, synthetic wastewater was fed to the reactors. The Suomenoja WWTP sludge
was gradually replaced by synthetic sludge created in the process.
The reactors had an operating volume of 12l and all four reactors operated
independently from each other. They were kept in a temperature controlled room at a
constant temperature of 12 ± 1°C. The temperature of 12°C was chosen because it is a
typical wastewater treatment temperature in Finland and because this is a possible limit
of nitrogen removal in the environmental permits of WWTPs. This is based on the
European Union wastewater directive. (The Commission of the European Communities,
One operating cycle lasted 8 hours, which meant a total of three cycles per day. The
pumps and the aeration were controlled by a timer. The timer is seen in Figure 4.
Figure 4 Picture of the timer that controlled the cycles in the reactors.
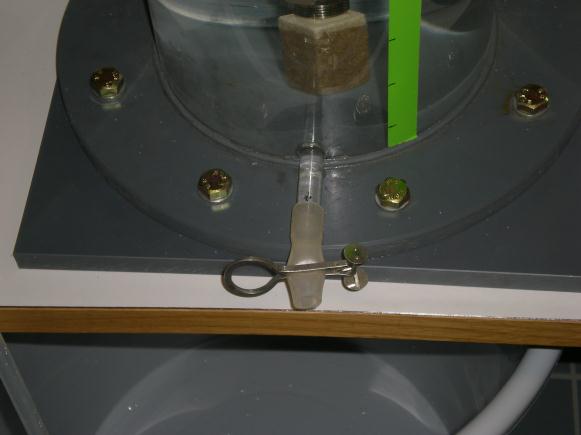
Every reactor had its own feeding pump and two reactors shared one draining pump. In
each cycle three liters of synthetic wastewater was added to the reactors and in the
decant stage an equal amount of cleaned water was removed.
Sludge was wasted manually through a flexible hose at the bottom of the reactor as seen
in Figure 5. A sludge age of 10 days was chosen because of a recommendation in the
POSEIDON report where it was stated that many PHCs biodegrade best in WWTPs
with SRTs of 10 days or more. (Ternes, 2004) This also enabled nitrification to take
place in 12°C. (Henze et al. 2002) The SRTaer meant that sludge was wasted
theoretically 750ml/day. In practice the sludge removal was manual and was removed
1875ml every Monday and Friday and 1500ml was removed every Wednesday. After
the aeration time was lengthened on 16.1.2012, the SRTaer was 12 days.
Figure 5 Pictures of the sludge wasting arrangement.
To provide oxygen and stirring, a ceramic aeration cube was placed in the bottom of
each reactor as seen in Figure 6. The aeration took place only during the react stage. No
additional mixture was needed because the aeration was enough to fully mix the tanks.
The air to the aeration stone was provided by a compressor. The amount of Dissolved
Oxygen (DO) was never under 4 mg/l for the entire cycle and during aeration the DO
was around 10mg/l. The amount of oxygen was not controlled.
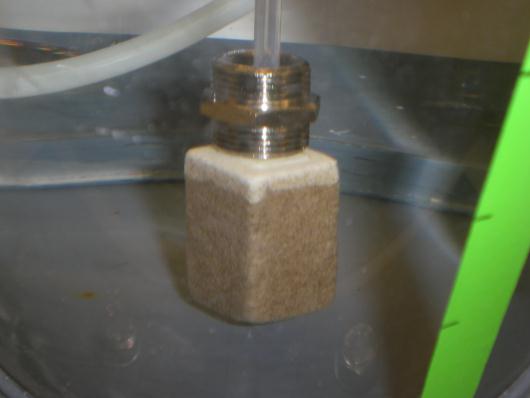
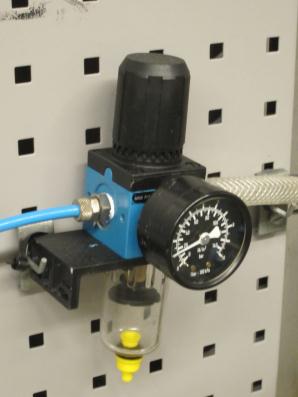
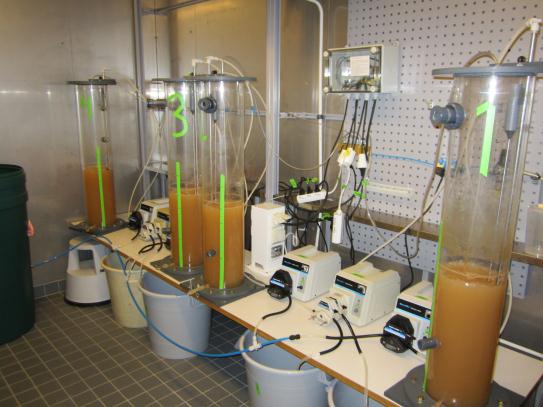
Figure 6 Pictures of the ceramic aeration cube in the bottom of the reactor and air rotameter.
Figure 7 Figure of the SBR arrangement.
Figure 8 Pictures of the SBR arrangement during aeration and settling.
The time for each stage in the SBR cycle was chosen based on a literary review. The
cycle with each stage can be found in
Figure 9 The process cycle from the beginning of the experiment to 13.1.2012.
The cycle was changed on 16.1.2012 due to problems with the operation. The second
cycle can be found inand more about the challenges of operating the pilot can
be found in Chapter 10.
Figure 10 The process cycle from 16.1.2012 to the end of the experiment.
Before starting the reactors, assessments and calculations were made to analyze
different scenarios. The settling of the sludge was seen as a risk. Preliminary
calculations were made of how different organics loads could influence the MLSS
concentration in the reactors. What that could have for consequences for the settling of
sludge, together with possible Sludge Volume Index (SVI) values, was also analyzed. It
was concluded that the organics load and composition in synthetic wastewater presented
in Chapter 9.4 would lead to stable operation of the reactors. As the estimations made
before the experiment varied from the actual values, the organics load was raised a
couple of weeks into the experiment. More about the organics load is presented in the
9.4 Synthetic wastewater
Synthetic wastewater was prepared two times a week, every three or four days. The
composition was the same as used in the master's thesis of Reetta Kuronen (2005). It
was based on the wastewater composition of the Suomenoja and Viikinmäki WWTPs in
Finland. (Kuronen, 2005)
The composition was changed after a couple of weeks of operation. The pH in the
reactors rose to 8.5 in the beginning of the experiment. Thus the amount of NaHCO3
was lowered twice; first the amount of NaHCO3 was lowered with 25% on 9.11.2011.
Since this was insufficient, the amount was lowered from the original concentration
with 43.75% on 25.11.2011. The amount of organic carbon was increased due to low
yields and low MLSS. The amount of peptone and yeast extract was increased with 80%
on 29.11.2011. After the changes, the pH in the reactors was 7.8 ± 0.2 and the MLSS
rose. The complete recipe of the wastewater after all the changes had been made can be
Table 15 Composition of synthetic wastewater after all the changes had been made
Composition of the synthetic wastewater added to the SBR (mg/l)
CH₃COONa * 3 H₂O
Yeast extract (Mereck 3753)
CaCl₂ * 2 H₂O
MgSO₄ * 7 H₂O
Nutrient solution
Composition of nutrient solution (g/l)
FeCl₃ * 6 H₂O
CuSO₄ * 5 H₂O
(NH₄)Mo₇O₂₄ * 4 H₂O
ZnSO₄ * 7 H₂O
CoCl₂ * 6 H₂O
In the beginning of the experiment, the average CODcr load was 320mg/l. When the
synthetic wastewater was made, the CODcr load was 350mg/l but due to reactions in the
synthetic wastewater tank, the composition of the water changed and the load decreased
over time. The sources of organics were peptone, yeast extract and sodium acetate.
Nutrients were also added to the wastewater. Nitrogen sources were yeast extract and
ammonia. The conditions in the beginning of the experiment can be found in
The HRT is calculated for the entire cycle.
Table 16 Conditions in the beginning of the experiment
Aerated share of cycle
g CODcr/ g MLVSS /d
g CODcr/ g MLSS /d
g BOD7(ATU)/ g MLVSS /d
g BOD7(ATU)/ g MLSS /d
The increase of peptone and yeast extract raised the CODcr and BOD7(ATU) loads. After
the change in the composition of wastewater, the average CODcr load was 470mg/l. And
the average BOD7(ATU) load was 370mg/l. The BOD7(ATU)/CODcr ratio was estimated to
On 16.1.2012 the reaction time and therefore the aeration was lengthened with 45
minutes. The conditions in the reactors, after all the changes in the process, can be
Table 17 Conditions in the end of the experiment
Aerated share of cycle
g CODcr/ g MLVSS /d
g CODcr/ g MLSS /d
g BOD7(ATU)/ g MLVSS /d
g BOD7(ATU)/ g MLSS /d
9.5 Storage solution of ibuprofen
A storage solution of ibuprofen was made by diluting 100mg of >98% ibuprofen
(purchased from Sigma-Aldrich) in 100ml of methanol and then adding ultrapure water
purified with reverse osmosis so that the stock solution had a total volume of 2l and an
ibuprofen concentration of 50mg/l. The stock solution was stored in the dark at +4°C.
Ibuprofen dissolved well in methanol, but after adding ultrapure water the solution
turned foggy and white. After adding more methanol and letting the solution stand
awhile the ibuprofen was again dissolved. However, before the third test some of the
ibuprofen was again precipitated. A new stock solution was prepared on 13.01.2012.
The second stock solution was stored and made in the same way as the first, with the
exception of that the ibuprofen was first dissolved in 100ml of methanol and after that
the ultrapure water was added. This time all the ibuprofen was dissolved and no
precipitation occurred after the addition of ultrapure water. Before the two last tests the
stock solution was taken to room temperature the day before and placed under mixing
for the night. This ensured that all the ibuprofen was properly diluted in the stock
9.6 Sampling and analyses
9.6.1 Weekly process analyses
Samples from the process were taken once a week in the beginning of the experiment
and every second week in the end of the experiment. Samples were analyzed to
determine how the process was working. The analyses that were made on a regular basis
are described i The results of the analyses can be found in Chapter 10. The
CODcr and MLSS analyses were made more often because these were good indicators
of how the process was working. Monitoring the organics removal in the reactors,
ensured that the process was working as planned and the MLSS showed that the sludge
was adapting well and producing new biomass. All analyses were made when the
system was spiked with ibuprofen. The sludge was also observed via microscope.
Table 18 Methods used in weekly analysis of conditions in the reactors
SFS-EN-ISO 11905-1 v.1998 + Standard Methods (v.2005)
Lange: Ganimede N
4500 B. Ultraviolet Spectrofotometric Screening Method +
Oxidation SFS-EN ISO 6878 v. 2004, measurment SFS-EN-
Foss: FIAstar 5000 Analyzer
ISO 15681-1, fia v. 2005
SFS-EN ISO 15681-1 v. 2005, tinchloride
Foss: FIAstar 5000 Analyzer
SFS-EN ISO 13395 v. 1997, fia
Foss: FIAstar 5000 Analyzer
SFS-EN ISO 13395, v. 1997
Foss: FIAstar 5000 Analyzer
ISO 11732 v. 2005
Tecator 5012 Analyzer, 5042 Detector
SFS-EN 872 v. 2005
SFS 5504 v. 1988WTW Applikationsbericht BSB 997 232 Respirometrische BSB₅-Bestimmung von mit hemmenden- oder
toxischen stoffen belastetes Abwasser mit dem OxiTop- Meßsystem
9.6.2 Ibuprofen analysis
The influent water of the SBRs was spiked four times with ibuprofen during the
experimental period. The spiking of influent were made on 30.11.2011, 19.12.2011,
25.1.2012 and 20.2.2012. The aims of the first test are listed below.
To determine the ibuprofen concentration in the storage solution, to see that the
preparation of the storage solution had been done accurately.
To analyze a sample of influent wastewater to ensure that it has the intended
concentration of ibuprofen. This was made to make sure that the correct
measurements had been made and that the compound is not lost somewhere
during the process.
To analyze one sample taken from a reactor during aeration to get a
preliminary indication of how and if the compound biodegrades.
The last three tests were made to determine a primary biodegradation rate and
biodegradation rate constant of ibuprofen in a pilot scale SBR. In the second test, one
reactor was left as a reference without spiked ibuprofen to ensure that no contamination
was taking place during the sample taking and preparing. The samples were sent to
another laboratory (Ramboll Analytics in Lahti) for analysis of ibuprofen. The results
can be found in Chapter 10.2.
The ibuprofen analysis made by Ramboll Analytics was done by the EPA Method 1694
Pharmaceuticals and Personal Care Products in Water, Soil, Sediment, and Biosolids by
HPLC/MS/MS. (United States Environmental Protection Agency, 2007) The error of
the analyses was estimated to be ca. 30% for both the sludge and water samples by the
The water samples with relatively high concentrations could be analyzed by diluting
straight to the right analyze interval. The water samples with low concentrations were
concentrated before the analysis. A known concentration of deuterized ibuprofen was
added to evaluate the yield of each analysis. The prepared sample was analyzed by
UPLC-MS/MS technology and quantified according to the yield of the known
The sludge samples, which were taken during aeration to determine the ibuprofen
concentration in the reactors, at a certain point of time, were filtered after throughout
mixing through a fiberglass filter (Schleicher & Schuell, GF 52 (Ref No. 10428230, 50
mm)). The solids were lightly rinsed with UHQ-water from the edges of the filter wall.
The solids with the filters were dried in room temperature. The solids were eluated by
putting them and a filter in a test tube, a known concentration of deuterized ibuprofen
was added to this. This was then extracted by a precise amount of methanol. The
extraction was made by first mixing the test tube for >60 minutes and then placing it in
an ultrasound container. After the extraction, some of the methanol was taken and
analyzed as the water samples. The ibuprofen in the sediment in the sludge samples
were added to the results obtained from the analysis of the water phase in the sample.
10. Results and discussion
10.1 Operation of reactors
The primary goals for the SBRs were for them to remove organics from synthetic
wastewater and nitrify. The four reactors were operated equally and the results show
that the reactors worked as designed. The analyses showed that all four reactors had
very similar organics and nutrient removals at all times. Nitrification was planned in the
process and also realized. More of the nutrient removal is described in Chapter 10.1.3.
The biggest change in the data occurred when the organics concentration in the
synthetic wastewater was increased. This change can be seen especially in the organics
and nitrogen concentrations in the reactors. A second change in the data can be
observed when the pumping of influent water was ceased for a weekend 13.1-15.1.2012
due to surface sludge, that is generally caused by denitrification, and the potential
problems that came with it. After this, it took some time for the process to recover.
The sludge was settling well. The SVI values stayed under 100ml/g during the entire
experimental period. This is a sign of a well settling sludge. (Metcalf & Eddy, 2003)
10.1.1 Biomass and activated sludge flocs
After the reactors were started, the sludge was first let to grow freely for one week.
Regular sludge removal was started after that. The sludge however had a very low yield
and under a microscope it was observed that very little microorganism were present in
samples. This together with a decreasing MLSS concentration indicated that the sludge
was not adapting well to the new surroundings. After lowering the pH on 25.11.2011
and increasing the organics load on 29.11.2011 the sludge seemed to start adapting
better. A higher MLSS concentration was analyzed a week later on 7.12.2011. The
change could also be observed via microscope where sludge samples had large clusters
of vorticella present. A picture of a cluster of vorticella is seen in
Figure 11 Picture of vorticella seen in a sample of well-adapted sludge via microscope 9.1.2012.
At the beginning of the experiment on 10.11.2011, the MLSS was 1.7g/l. This
decreased to 1g/l due to low loads. When the organics load was increased, the MLSS
rose to a level of 1.6g/l. The share of Volatile Suspended Solids (VSS) was in the
beginning 75%. This amount increased during the experiment to almost 90% and was
probably due to the lowered iron concentration in the sludge. The average MLSS and
MLVSS concentrations are illustrated in Figure 12.
Figure 12 The average of MLSS and MLVSS in the reactors during the experimental period. (The individual
values can be found in appendix 1)
The amount of SS in effluent wastewater was below 20mg/l even at the highest, which
indicated that no large amount of sludge was escaping from the SBR with the effluent
water. The average SS in the effluents are illustrated in Figure 13.
Figure 13 The average SS concentration in effluent water. (The individual values can be found in appendix 1)
The total iron concentration in the sludge was analyzed regularly to detect how much of
the original sludge had been replaced with the artificially grown sludge. As the sludge
used in the start of the process was collected from Suomenoja WWTP, where iron is
used for chemical phosphorus removal, the decreasing iron content was a good
indication that the process had started producing new biomass and that the amount of
Suomenoja WWTP sludge was gradually decreasing in the reactors. The total iron
concentration decreased from over 120mg/l to 0.9mg/l during the experimental period
and this is illustrated in Figure 14.
Figure 14 The average total iron concentration in the reactors. (The individual values can be found in
appendix 1)
10.1.2 CODcr and BOD7(ATU)
The CODcr in both the influent and effluent water was measured regularly throughout
the experimental period. The CODcr and the BOD7(ATU) in the influent wastewater
depended somewhat on the difference in days when the synthetic wastewater was
prepared and the day of sampling. The BOD7(ATU) in the influent and effluent
wastewater was measured on two occasions at the end of the experiment to ensure that
the BOD7(ATU) removal was sufficient and in line with the CODcr removal.
The CODcr of the effluent wastewater was mainly between 50-100mg/l with an average
of 89mg/l. This seemed to mostly depend on the influent CODcr load. The CODcr
removal rates varied from 74-90% with an average of 83% for the entire experimental
period. The CODcr removal is illustrated in Figure 15.
Figure 15 The average CODcr removed. (Individual values of CODcr in influent and effluents can be found in
appendix 1)
The BOD7(ATU) of the effluent water was analyzed on 3.2.2012 and 14.2.2012. At the
time of samplings, the effluent waters had a CODcr concentration of 50mg/l. The
average BOD7(ATU) concentrations in the effluent wastewaters were 8mg/l. The
BOD7(ATU) removal rates were about 96%.
10.1.3 Nutrient removal
Nutrient removal was not the primary goal when designing the SBRs. Both nitrogen and
phosphorus were removed to some extent in the process. The removal could be mostly
contributed to assimilation due to the microorganism growth. The removal rate for
phosphorus varied between 15% and 40%. The phosphorus removal did not seem to be
effected by the decreasing concentration of iron in the sludge or suspended solids
concentration in the effluents. The average phosphorus removal is illustrated in
Figure 16 Average total phosphorus removed.
All of the phosphorus in the effluent wastewater was present as phosphate. The
phosphate was analyzed as soluble phosphate and the sample was filtered through a
0.45µm membrane. The phosphorus and phosphate concentrations in influents and
effluents are illustrated in
Figure 17 Average phosphorus and phosphate in influent and effluents. (Individual values can be found in
appendix 1)
The total nitrogen removal was more even than the phosphorus with removal of 30-
45%. The total nitrogen removal is illustrated in Even though the nitrogen
load increased as the amount of carbon load was raised, the nitrogen removal stayed on
the same level. The phosphorus and nitrogen concentrations in sludge are illustrated in
Figure 18 The Average Ptot and Ntot concentrations in reactors. (Individual values can be found in appendix 1)
Figure 19 Average total nitrogen removed.
Ammonium was reduced in the reactors with indicates that the process was nitrifying
even in the beginning of the experiment. All four reactors were nitrifying perfectly and
the nitrification rate was over 99% through the entire experimental period. The
ammonium concentration, together with the total nitrogen concentration in influents and
effluents are illustrated in
Figure 20 Average total nitrogen and ammonium in influent and effluents. (Individual values can be found in
appendix 1)
The amount of NO2 was measured on 8.2.2012 to ensure that most of the NO3+NO2 was
nitrate. It was observed that 98% of the measured NO3+NO2 concentration was nitrate.
The NO3+NO2 concentration through the experimental period is presented in
Figure 21 Average NO3+NO2 in effluents. (Individual values can be found in appendix 1)
10.1.4 Denitrification
On 12.1.2012 the tubing of the influent wastewater feeding system was changed. There
was some precipitate or biofilm formation in the tubes and to ensure that the
concentration of the marker compound would not change, the tubing was changed.
However, this obviously lead to a slightly increased organics load and the sludge was
noticed to denitrify and rise up to the surface at the end of the cycle. A picture of the
surface sludge is seen in Figure 22. There was a high risk that the sludge would
accidentally be removed from the reactors during the decant stage. This was observed
on 13.1.2012. The pumping of influent wastewater was ceased as this was observed on a
Friday afternoon and aeration was put on for the weekend. On Monday 16.1.2012 the
process was started again with a lengthened aeration period and a shortened settling
time. The aeration time was increased with 45 minutes and settling time decreased
respectively. This way the reactors were given more time to use the total organics load
fed into the system. Also the settling time, which made possible the anoxic conditions
causing denitrification, was shortened.
The feeding tubes were again changed on 15.2.2012 prior to the last test. This time
surface sludge was also observed in the reactors. This time, however, no additional
steps were taken to hinder this, as the problem was not as severe as before. This meant
that no risk of the sludge to be accidentally removed from the reactors during the draw
stage was expected. Surface sludge was not observed after the process had adapted to
the new conditions.
Figure 22 Picture of the sludge raised to surface due to denitrification on 13.1.2012.
10.2 Ibuprofen
10.2.1 The first spiking of ibuprofen
The first wastewater spiked with ibuprofen was added to the process on 30.11.2011. A
volume of 20ml stock solution was added to 50l of synthetic wastewater so that the final
calculated ibuprofen concentration added to the reactors was 20µg/l. This meant that the
calculated ibuprofen concentration in the reactors at the beginning of the aeration stage
would be 5µg/l.
A sample of the stock solution, spiked synthetic wastewater and sludge were sent to
Ramboll Analytics to be analyzed. The sludge sample was removed from reactor 1, 200
minutes after the start of the aeration stage. The samples of sludge and synthetic
wastewater were preserved by adding HCl to lower the pH to 3. The samples were
stored in +4°C until analysis.
The results from the first test can be found in The amount of ibuprofen in the
storage solution was as intended 50mg/l. Also the amount of ibuprofen in the influent
wastewater was analyzed to be 24µg/l ± 30% so the calculated concentration of 20µg/l
fits within this margin. Calculating from this, the initial amount of ibuprofen in the
beginning of the aeration was 6µg/l. The initial sludge sample indicated that the
compound biodegrades fast in the reactor, up to 98% in 200 minutes. Although not
enough data was obtained to further determine the biodegradation of ibuprofen, this test
ensured that spiking and sampling procedures were done accurately enough.
Table 19 Results from the first spiking of ibuprofen on 30.11.2011
Influent
10.2.2 The second spiking of ibuprofen
The second spike of ibuprofen was added to the pilot on 19.12.2011. During the second
test, all changes to the synthetic wastewater had been made, see Chapter 9.4. Reactor 2
was not spiked with the compound, but left for control to ensure that no contamination
was taking place during testing, sample preparation and analysis. A volume of 100ml
stock solution was added to 50l synthetic wastewater. The target concentration of
ibuprofen in the influent water was therefore 100µg/l and the calculated concentration
in the reactors was 25µg/l at the beginning of the aeration. Samples of both the spiked
and not spiked synthetic wastewaters were sent for analysis. Also four sludge samples,
from reactor 1, taken at different times, and a sample of effluent were sent for analysis.
A sludge sample from reactor 2 was also analyzed to check for contamination. All
samples were preserved by lowering the pH to 3 with HCl and stored in +4°C until
The results from the second adding of ibuprofen to the reactor can be found in
an This time the influent wastewater sample was taken directly from the
feeding tube and this might have been a reason for why the ibuprofen concentration was
lower in the second test. Some of the compound might have got stuck or already
biodegraded in biofilm and other accumulations in the feeding tubes. Totally 65% of the
added ibuprofen was caught in the influent wastewater when analyzed. This meant that
the starting concentration in the SBR reactor was 16µg/l ± 30% instead, of the
calculated 25µg/l.
The sludge samples, taken at different times in the aeration period, indicate that the
ibuprofen biodegrades in the reactors, but not as much as the first test showed. A
significant concentration of the compound was also found in the effluent wastewater
which means that the reactor did not biodegrade all of the ibuprofen but only around
53% of the initial concentration. The control samples did not contain ibuprofen which
means that no contamination was taking place during the testing, sampling or analysis.
A specific biodegradation rate was calculated to be 1.6µg ibuprofen/gVSS/h.
Table 20 Results from the second test on 19.12.2011
Added and analysed concentration of ibuprofen in influent water
Influent (control)
Analysed concentrations of ibuprofen in reactors
Minutes into the
Concentration in
Concentration in
reactor 1 (µg/l)
reactor 2 (µg/l)
Ibp concentration
in effluent water
Figure 23 Ibuprofen concentration change in reactor 1 on 19.12.2011.
10.2.3 The third spiking of ibuprofen
The third test was made on 25.1.2012. By this time all changes to the process had been
made and the conditions in the SBRs can be found in This time all four
reactors were spiked with the same amount of ibuprofen. This way a more reliable
statistical evaluation of the biodegradation could be calculated. Synthetic wastewater
spiked with 100µg/l ibuprofen was added to all four reactors. Two influent water
samples were sent for analysis. One of the influent water samples was taken at the end
of the feeding tube and the other directly from the synthetic wastewater tank. All in all
16 sludge samples and 4 effluent water samples were also sent for analysis. All samples
were preserved by lowering the pH to 3 with HCl and stored in +4°C until analysis.
The complete results from the third test can be found in Table 21 and Figure 24. The
influent wastewater sample was taken directly from the feeding tube of reactor 2. This
meant that influent water was manually added to the reactor 2. The four sludge samples
were taken at the same times as in the second test.
This time the yield of ibuprofen was over 90% of the added amount in the synthetic
wastewater, in the influent wastewater analysis. It was however noted that the error in
analysis is most likely larger than the ones that result from the spiking procedures, so
the calculated influent concentration of 100µg/l was used in all further calculations. As
the tubing had been changed before the test, no ibuprofen was believed to be stuck in
biofilm in the tubes.
The sample taken 15 minutes after the beginning of aeration was not considered in the
calculation of biodegradation rate constant. The results indicate that the compound had
not, at this point, been evenly mixed in the tank, which made this data unreliable.
The changes in the ibuprofen concentrations are similar in all reactors. The
biodegradation that took place, varied from 52-56%, where reactor 1 had the lowest
removal. The degraded amount of the compound is similar to detected amount in the
second test, where the degradation was about 53%. The specific biodegradation rate was
calculated to be 1.9, 2.1, 1.5 and 1.5µg ibuprofen/gVSS/h for reactors 1 ,2 ,3 and 4
Table 21 Results from the third test on 25.1.2012
Added and analysed concentration of ibuprofen in influent water
Influent in reactor 2
Influent in reactors 1, 3 and 4
Analysed concentrations of ibuprofen in reactors
Minutes into the
Concentration in
Concentration in
Concentration in
Concentration in
reactor 1 (µg/l)
reactor 2 (µg/l)
reactor 3 (µg/l)
reactor 4 (µg/l)
Ibp concentration
in effluent water
Figure 24 Ibuprofen concentration change in all four reactors on 25.1.2012.
10.2.4 The fourth spiking of ibuprofen
The fourth adding of ibuprofen in the reactors was made on 20.2.2012. The goal in this
test was to repeat the experiment made last time and verify the data. This time analyses
were only made of two reactors, reactors 1 and 2. The influent water sample was taken
from the end of reactor 3 feeding tube. All tubing was changed before the experiment to
ensure that ibuprofen would not react or sorb due to the biofilm in the feeding tubes.
Also, to save analysis costs, only 3 sludge samples from each reactor were sent for
analysis. The samples from the reactors were taken at 60, 180 and 300 minutes after the
beginning of aeration.
As in the previous test, the synthetic wastewater was spiked with ibuprofen so that the
calculated concentration was 100µg/l in the influent water and 25µg/l in the reactors at
the beginning of aeration. As in the third test, the calculated concentration in the
influent was used in the further calculations of biodegradation rate and biodegradation
rate constant. In the fourth test the biodegradation rate seemed to be lower than in the
previous ones. The compound degraded 36% in reactor 1 and 28% in reactor 2. The
results from the fourth test are presented in and The specific
biodegradation rate was calculated to be 0.9µg ibuprofen/gVSS/h for both reactors.
Table 22 Results from the test on 20.2.2012
Added and analysed concentration of ibuprofen in influent water
Analysed concentrations of ibuprofen in reactors
Minutes into the
Concentration in
Concentration in
reactor 1 (µg/l)
reactor 2 (µg/l)
Ibp concentration
in effluent water
Figure 25 Ibuprofen concentration change in reactor 1 and reactor 2 on 20.2.2012.
10.2.5 Biodegradation of ibuprofen
According to the literary review presented in Chapter 4.3.1 it was expected that
ibuprofen would biodegrade. The biodegradation and biodegradation rate constant were
determined as explained in Chapter 3.3.1. The biodegradation of ibuprofen is seen as a
pseudo first order degradation. In the first test, enough data was not obtained to
determine a biodegradation rate and biodegradation rate constant. In the second, third
and fourth tests, these could be calculated. As mentioned in Chapter 10.2.3, the
sampling point at 15 minutes after the beginning of aeration was not considered in the
calculations of the constant.
As the biodegradation of the compound was seen as a pseudo first order degradation,
where the degradation depended on the amount of MLSS in the reactors, the
concentrations of ibuprofen in the reactors (as ln(C0/Ca)) were plotted as a function of
time. The plots are presented in Figures 26, 27 and 28. A linear trend line was added to
the plot. The slope k´ of the trend line was divided by the MLSS concentration in the
reactor to obtain the biodegradation rate constant kbiol.
Figure 26 Trend line from the second test 19.12.2011.
Figure 27 Trend lines from the third test 25.1.2012.
Figure 28 Trend lines from the fourth test 20.2.2012.
From the obtained kbiol values from each test, an average constant was calculated that
describes the biodegradation of ibuprofen in the SBR in the temperature of 12°C. A
summary of the gained constants is presented in The average biodegradation
rate constant with the minimum, maximum and standard deviation is presented in
The average specific biodegradation rate is presented in Table 25.
Table 23 Summary of the biodegradation rates and biodegradation rate constants obtained in the study
Specific biodegradation rate
MLSS (g/l)
Biological transformation (µg ibuprofen/gVSS/h)
Table 24 The average biodegradation rate constant with standard deviation and minimum and maximum
values
Table 25 Average specific biodegradation rate obtained in the study with standard deviation and minimum
and maximum values
Specific biodegradation rate
10.2.6 Comparing the results to literature
Values found in literature for kbiol were a bit higher than the ones obtained in this study.
This could be a result of many different factors. As studies do not contain all the
background information needed to compare the differences, no conclusive comparison
can be made. In the study made by Joss et al. (2006) enough information was available
to make some speculations. The study is presented in Chapter 4.3.1 and a summary of
the conditions in that experiment and this, is presented in Table 26. In the study they
obtained the highest biodegradation rates, the MLSS was over double that of this study.
Also the influent water had a lower CODcr load and the temperature was higher.
Table 26 Summary of the conditions in experiments made by Joss et al. (2006) and this study
MLSS (g/l) COD (mg/l)
Joss et al. (2006)
As Clara et al. (2005) concluded that the temperature should not have big effect on the
biodegradation of ibuprofen, it is more likely that the SRT and organics load had a
larger effect on the results. This could also explain the big difference between the
results obtained in the first and three last tests. For conclusive answer to this question,
more study is needed. Another factor that seemed to influence the biodegradation was
the MLSS concentration.
Another factor that could have influenced the lower biodegradation rate constants was
that the sludge in this study was not accustomed to ibuprofen when it was spiked with
the compound. In the other studies that have been presented the sludge was accustomed
11. Conclusions
Potentially harmful compounds are a growing interest among researchers. While it has
been known since the last century that POPs cause damage to the environment, new
emerging pollutants are being constantly detected. Many man-made compounds have
been found to have endocrine disruptive tendencies and to pose a threat to the
reproductive systems of animals. As many of the PHCs are present in household
products such as plastic, electronics and personal care products, the exposure to these
happens mostly before ending up in waters via WWTPs. To control their distribution at
source and forbid their use seems like a safer and environmentally friendlier option.
Pharmaceuticals are a different challenge as, contrary to other PHCs, their use is hard to
restrict and replace with other compounds. Other ways to reduce their presence and
threat to the environment needs to be studied. A special threat is antibiotics and anti-
microbial compounds as their presence in the environment increase the risk of antibiotic
resistant bacteria being formed.
Biodegradation is the most preferable way of removing PHCs from wastewater because
this is the only way of ensuring that they do not end up in the environment. Problems
arise when PCHs sorb to sludge and the sludge is used in agriculture and other ways
relocated into the environment.
Measuring and analyzing compounds at concentrations of µg/l is challenging. The
analyzing equipment and procedures will hopefully improve in the future and make this
sort of study easier to a wide range of compounds. A lot is still unknown about the
microorganisms and conditions behind biodegradation. This results in that it is not
known if and what advanced treatments are needed to keep the environment safe from
harmful compounds. Activated sludge, when process conditions are optimized, is a
strong candidate for obtaining good results in PHC-removal. The cold conditions that
are present in Finnish WWTP may slow the biodegradation of certain compounds. To
understand the fate of PHCs in the special conditions that prevail in the Nordic
countries more study is needed.
In this study, it could be determined that ibuprofen is biodegraded in SBRs without an
adaptation period. The first test made in this research implied that ibuprofen was
biodegraded up to 98%, while the future tests implied that only around 30-50% is
biodegraded. The cause of this is not known. A possibility however is that while the
SBRs had a lower organics load in the first test than in the rest, the process could better
use up the ibuprofen.
The biodegradation rate constants obtained in the SBRs are a bit lower than those found
in literature. This can be a result of a number of things. The SBRs were working in cold
temperature, had a sludge age of only 12 days and the reactors were not accustomed to
ibuprofen as it was not constantly fed into the system. The reactors had also a high F/M-
ration. A weakness of the biodegradation rate constant is that it only is valid for the
specific conditions where it is determined. But it gives a good indication of the
biodegradation potential. Literature values are hard to compare with each other as they
are obtained in very different ways and all test conditions are not fully documented. A
big uncertainty in the results was the margin of error in the analysis. As it was so large,
exact concentrations were not known. Hopefully the analysis will in future be more
precise and exact biodegradation rates and constants could be determined.
If ibuprofen was mineralized or turned into other compounds was not studied in the
experiments. So it is not known if the compound is really biodegraded or if its
metabolites are let to nature where they can metabolize to the parent compound. It was
also not studied if the biodegradation of ibuprofen was through co-metabolism or
catabolic metabolism. Most studies only analyze one compound at a time. Knowledge
of synergy effects and actual behavior of different compounds in mixtures are scarce.
Synergy effects can have huge implication on compounds behavior in WWTPs and the
harmful effects to the environment.
11.1 Future research areas
There are many questions to be answered to better understand the effects of PHCs in the
environment and how these can be reduced in WWTPs. How and by which
microorganisms different compounds are degraded can give a better insight to what
kind of conditions are needed in WWTPs to optimize the biodegradation. Another way
to determine the optimal WWTP conditions for PHC-removal would be to study how
SRT, temperature and organics load effect biodegradation of a compound. This could be
studied in both conventional activated sludge and more advanced treatments. By
comparing the difference in biodegradation, costs and environmental impacts, the use of
advanced treatments could better be considered. Using and building more advanced
treatments to WWTP can results in extremely high costs and increased greenhouse gas
emissions. The benefits of reducing the concentrations of PHCs by advanced treatments
may be overshadowed by the negative impacts of implementing them.
As many of the compounds are poorly biodegradable and sorb to sludge, the sludge
treatment and the possibilities there to reduce the amount of PHCs there would be
important to study. This could ensure safer sludge disposal and reuse.
Another interesting aspect is the rising concern over antibiotics in WWTPs and the
environment. An interesting question to study would be how antibiotics impact the
bacterial community in WWTPs. Do they alter the bacterial community in any way and
does antibiotic resistance in bacteria increase in WWTPs where antibiotics are present?
Also the threat antimicrobial compound like triclosan might impose in reference to
antibiotic resistant bacteria is an unanswered question.
The amount of pharmaceuticals in the effluents from pharmaceutical factories has not
traditionally been seen as a large source of pharmaceuticals. Bigger interests have been
municipal WWTPs and hospital wastewaters. An interesting research area would be to
study the effluents from pharmaceutical factories. This can be very important to study,
especially in countries where wastewaters from industries are not properly treated.
References
Albrecht, M. A., Evans, C. W. & Raston, C. L., 2006. Green chemistry and the health
implications of nanoparticles. Green Chemistry , Volume 8, pp. 417-432.
Batt, A. L., Kim, S. & Aga, D. S., 2006. Enhanced Biodegradation of Ioromide and
Trimethroprim in Nitrifying Activated Sludge. Environmental Science Technology ,
Volume 40, pp. 7367-7373.
Batt, A. L., Kostich, M. S. & Lazorchak, J. M., 2008. Analysis of Ecologically Relevant
Pharmaceuticals in Wastewater and Surface Water Using Selective Solid-Phase
Extraction and UPLC-MS/MS. Analytical Chemistry , Volume 80, pp. 5021-5030.
Beruetta, L., Gallo, B. & Vicente, F., 1995. A Review of Solid Phase Extraction: Basic
Principles and New Developments. Chromatographia , Volume 40, pp. 474-483.
Bitton, G., 2005. Wastewater Microbiology. 3rd ed. New Jersey: Jhon Wiley & Sons.
Bolong, N., Ismail, A., Salim, M. & Matsuura, T., 2009. A review of the effects of
emerging contaminants in wastewater and options for their removal. Desalination ,
Volume 239, pp. 229-246.
Brorström-Lundén, E, Svenson, A, Viktor, T, Woldegiorgis, A, Remberger, M, Kaj, L,
Dye, C, Bjerke, A & Schlabach, M 2008. Measurments of Sucralose in the Swedish
Screening Program 2007 -Part I', IVL Report B1769, Swedish Environmental Research
Institute, Stockholm.
Carballa, M., Omil, F. & Lema, J. M., 2007. Calculation Methods to Perform Mass
Balances of Micropollutants in Sewage Treatment Plants. Application to
Pharmaceuticals ans Personal Care Products (PPCP). Enviromental Science and
Technology, Volume 41, pp. 884-890.
Chen, X, Lund Nielsen, J, Furgal, K, Liu, Y, Lolas, IB & Bester, K 2011.
Biodegradation of triclosan and formation of methyl-triclosan in activated sludge under
aerobic conditions. Chemosphere, Volume 84, pp. 452-456.
Clara, M, Strenn, B, Gans, O, Martinez, E, Kreuzinger, N & Kroiss, H 2005. Removal
of selected pharmaceuticals, fragrances and endocine disrupting compounds in a
membrane bioreactor and conventional wastewater treatment plants. Water Research,
Volume 39, no. 19, pp. 4797-4807.
Combalbert, S. & Hernandez-Raquet, G., 2010. Occurance, fate, and biodegradation of
estrogens in sewage and manure. Applied Microbiology Biotechnology , Volume 86, pp.
Det Norske Veritas, 2006. Kartlegging av utvalgte forbindelser i legemidler og
kosmetikk, Oslo: Det norske Veritas.
Bisphenol
[Accessed 20 12 2011].
European Union-Risk Assesment Report, 2008. 1,3,4,6,7,8-HEXAHYDRO-4,6,6,7,8,8-
[Accessed 4 October 2011].
Farré, . M., Petrovic, M. & Barceló, D., 2007. Recently developed GC/MS and LC/MS
methods for determining NSAIDs in water samples. Analytical Bioanalytical Chemistry,
Volume 387, pp. 1203-1214.
Fatta-Kassinos, D., Meric, S. & Nikolaou, A., 2011. Pharmaceutical residues in
environmental waters and wastewater: current state of knowledge and future research.
Analythical Bioanalythical Chemistry , Volume 399, pp. 251-275.
Federle, T. W., Kaiser, S. K. & Nuck, B. A., 2002. Fate and effects of triclosan in
activated sludge. Environmental Toxicology and Chemistry , Volume 21, pp. 1330-
Finnish Medicines Agency, 2011. Lääkekulutus vuosina 2007 - 2010, Helsinki
Finnish Ministry of Environment, 2010. Miten nanohiukkaset käyttäytyvät joutuessaan
luontoon?.
[Accessed 21 12 2011].
Finnish Ministry of the Environment, 2005. Vesiympäristölle haitalliset ja vaaralliset
aineet pintavesissä, Helsinki: Edita Prima Oy.
Finnish Ministry of the Environment, 2010. Bromatut palonestoaineet. [Online]
[Accessed 28 10 2011].
Suomenojan
prosessikaavio.
vio_suomenoja.pdf
[Accessed 21 12 2011].
Henze, M., Harromöes, P., la Cour Jansen, J. & Arvin, E., 2002. Wastewater Treatment:
Biological and Chemical Processes. 3rd ed. Berlin: Springer-Verlag.
Johnson, AC, Aerni, -R, Gerritsen, A, Gibert, M, Giger, W, Hylland, K, Juergens, M,
Nakari, T, Pickering, A, Suter, MJ-F, Svenson, A & Wettstein, FE 2005. Comparing
steroid estrogens, and nonylphenol content across a range of European plants with
different treatment and management practicies. Water Research, Volume 39, pp. 47-58.
Jones, O. A., Green, P. G., Voulvoulis, N. & Lester, J. N., 2007. Questioning the
Excessive Use of Advanced Treatment to Remove Organic Micropollutants from
Wastewater. Environmental Science and Technology, Volume 41, pp. 5085-5089.
Jones, O. A. H., Volvoulis, N. & Lester, J., 2005. Human Pharmaceuticals in
Wastewater Treatment Processes. Environmental Science and Technology, Volume 35
Joss, A, Zabczynski, S, Göbel, A, Hoffman, B, Löffler, D, McArdell, CS, Ternes, TT,
Thomsen, A & Siegrist, H 2006. Biological degradation of pharmaceuticals in
municipal wastewater treatment: Proposing a classification scheme. Water Research,
Volume 40, pp. 1686-1696.
Khan, S., Mukherjee, A. & Chandrasekaran, N., 2011. Silver nanoparticles tolerant
bacteria from sewage environment. Journal of Environmental Sciences , Volume 23, pp.
Kidd, KA, Blanchfield, MH, Palace, PV, Evans, RE, Lazorchak, JM & Flick, RW 2007.
Collapse of a fish population after exposure to a syntetic estrogen. PNAS Volume 104
No 21, pp. 8897-8901
Kim, D., Park, C.-S., Murayama, M. & Hochella, M. F. J., 2010. Discovery and
characterization of silver sulfide nanoparticles in final sewage sludge products.
Environmental Science & Technology , Volume 55, pp. 7509-7514.
Kim, S. & Aga, D. S., 2007. Potential Ecological and Human Health Impacts of
Antibiotics and Antibiotic-Resistant Bacteria From Wastewater Treatment Plants.
Journal of Toxicology and Environmental Health, Volume 10, pp. 559-573.
Korkki, K., 2006. Perfluorattujen alkyyliaineiden (PFAS) aiheuttamat ympäristöriskit
Suomessa, Helsinki: Edita Prima Oy.
Koskinen, P, Silvo, K, Mehtonen, J, Ruoppa, M, Hyytiä, H, Silander, S & Sokka, L
2005. Esiselvitys tiettyjen haitallisten orgaaniste aineiden päästöistä, Suomen
Ympäristökeksus, Helsinki.
Kraigher, B, Kosjek, T, Heath, E, Kompare, B & Mandic-Mulec, I 2008. Influence of
pharmaceutical residues on the structure of avtivated sludge bacterial communities in
wastewater treatment bioreactors. Water Research, Volume 42, pp. 4578-4588.
Kummerer, K., Menz, J., Schubert, T. & Thiemelans, W., 2011. Biodegradability of
organic nanoparticles in the aqueous environment. Chemosphere , Volume 82, pp.
Kupper, T, Plagellat, C, de Alencastro, LF, Grandjean, D & Tarradellas, J 2006. Fate
and removal of polycyclic musks, UV filters and biocides during wastewater treatment.
Water Research, Volume 40, pp. 2603-2612.
Kuronen, R., 2005. Enhancing wastewater nitrogen removal in activated sludge process
by using natural zeolites, Espoo
Larsson, J., de Pedro, C. & Paxeus, N., 2007. Effluent from drug manufacturers
contains extremely high levels of pharmaceuticals. Journal of Hazardous Materials ,
Volume 148, pp. 751-755.
Lehtonen, A., 2011. Changes in microbial diversity of biological carrier reactor
removing organic detrimental elements, Espoo
Lindberg, RH, Wennberg, P, Johansson, MI, Tysklind, M & Andersson, BA 2005.
Screening of Human Antibiotic Substances and Determination of Weekly Mass Flows
in Five Sewage Treatment Plants In Sweden. Environmental Science & Technology
Volume 39, pp. 3421-3429.
Lischman, L, Smyth, SA, Sarafin, K, Kleygwegt, S, Toito, J, Peart, T, Lee, B, Servos,
M, Beland, M & Seto, P 2006. Occurence and reductions of pharmaceuticals and
personal care products and estrogens by municipal wastewater treatment plants in
Ontario, Canada. Science of the Total Environment, Volume 367, pp. 544-558.
Liu, Z.-h., Kanjo, Y. & Mizutani, S., 2009. Removal mechanisms for endorcine
disrupting compounds (EDCs) in wastewater treatment-physical means, biodegradation,
and chemical advanced oxidation: A review. Science of the Total Environment, Volume
407, pp. 731-748.
Loos, R, Gawlik, BM, Boettcher, K, Locoro, G, Contini, S & Bidoglio, G 2009.
Sucralose screening in European surface waters using a solid-phase extraction-liquid
chromatography–triple
Chromotography A, Volume 1216, pp. 1126-1131.
Mace, S. & Mata-Alvarez, J., 2002. Utilization of SBR Technology for Wastewater
Treatment: An Overwiew. Industria &l Engineering Chemistry Research, Volume 41,
Malmi, H. & Mannio, J., 2008. Haitallisten aineiden näytteenotto ja esiintyminen
jätevedenpuhdistamoilla, Helsinki: Suomen Ympäristökeskus.
Marttinen, S. K., Kettunen, R. H., Sormunen, K. M. & Rintala, J. A., 2003. Removal of
bis(2-ethylhexyl) phthalate at a sewage treatment plant. Water Research , Volume 37,
Maurer, M, Escher, BI, Richle, P, Schaffer, C & Adler, AC 2007. Elimination of β-
blockers in sewage treatment plants. Water Research, Volume 41, pp. 1614-1622.
Metcalf & Eddy, 2003. Wastewater Engineering; Treatment and Reuse. 4th ed. New
York: McGraw Hill.
Mueller, N. C. & Nowack, B., 2008. Exposure modelling of engineered nanoparticles in
the environment. Environmental Science & Technology , Volume 42, pp. 4447-4453.
New England Interstate Water Pollution Control Commission, 2005. Sequencing Batch
Reactor Desing and Operational Considerations, Massachusetts
Paxéus, N., 2004. Removal of selecten non-steroidal anti-inflammatory drugs
(NSAIDs), gemifibrozil, carbamazepine, beta-blockers, trimethroprim and triclosan in
conventional wastewater treatment plants in five EU countries and their discharge to the
aquatic environment. Water Science and Technology , Volume 50, pp. 153-260.
Racz, L. & Goel, R. K., 2010. Fate and removal of estrogens in municipal wastewater.
Journal of Environmental Monitoring,Volume 12, pp. 58-70.
Radjenovic, J., Petrovic, M. & Barcelo, D., 2009. Fate and distribution of
pharmaceuticals in wastewater and sewage sludge of the concventional activated sludge
(CAS) and advanced bioreactor (MBR) treatment. Water Reasearch , Volume 43, pp.
Rang, H. P., Dale, M., Ritter, J. & Moore, P., 1995. Pharmacology. 5th ed. Loanhead:
Roh, H, Subramanya, N, Zhao, F, Yu, C-P, Sandt, J & Chu, K-H 2009. Biodegradation
ammonia-oxidizing
Chemosphere,Volume 77, pp. 1084-1089.
Roslev, P, Vorkamp, K, Aarup, J, Fredriksen, K & Nielsen, PH 2007. Degradation of
phthalate esters in an activated sludge wastewater treatment plant. Water Research,
Volume 41, pp. 969-976.
RPA, 2005. Risk assessment studies on targeted consumer applications of certain
organotin compounds, Norfolk: RPA.
Sáez , M., de Voogt , P. & Parsons, J. R., 2008. Persistence of perfluoroalkylated
substances in closed bottle test with municipal sewage sludge. Environ Sci Pollut Res
15, pp. 474-477.
Scott Fogler, H., 2009. Elements of Chemical Reaction Engineering. Westford: Pearson
Shaar, H., Clara, M., Gans, O. & Kreuzinger, N., 2010. Micropollutants removal during
biological wastewater treatment and a subsequent ozonation step. Environmental
Pollution , Volume 158, pp. 1399-1404.
Sinclair, E. & Kannan, K., 2006. Mass Loading and Fate of Perfluoroalkyl Surfactants
in Wastewater Treatment Plants. Environmental Science & Technology , Volume 40,
Smook, T., Zho, H. & Zytner, R. G., 2008. Removal of ibuprofein from wastewater -
comparing biodegradation in conventional, membrane bioreactor and biological nutrient
systems. Water Science & Technology, Volume 57, pp. 1-8.
Soares, A, Guieysse, B, Jefferson, B, Cartmell, E & Lester, JN 2008. Nonylphenol in
the environment: A critical review on occurence, fate, toxicity and treatment in
wastewaters. Environment International, Volume 34, pp. 1033-1049.
Soh, L., Connors, K. A., Brooks, B. W. & Zimmerman, J., 2011. Fate of Sucralose
through Environmental and Water Treatment Processes and Impact on Plant Indicator
Species. Environmental Science and Technology , Volume 45, pp. 1363-1369.
Solen, K. A. & Harb, J., 2011. Introduction to Chemical Engineering; Tools for Today
and Tomorrow. 5th ed. Hoboken, NJ: Wiley.
Stasinakis, A. S., Thomaidis, N. S., Nikolaou, A. & Kantifes, A., 2005. Aerobic
biodegradation of organotin compounds in activated sludge batch reactors.
Environmental Pollution , Volume 134, pp. 431-438.
Stockholm Convention, 2009. Supporting document for the risk profile on short-
[Accessed 19 January 2012].
[Accessed 28 October 2011].
Suárez, S., Carballa, M., Omil, F. & Lema, J. M., 2008. How are pharmaceuticals and
personal care products (PPCPs) removed from urban wastewaters?. Reviews in
Environmental Science and Biotechnology , Volume 7, pp. 125-138.
Swedish Environmental Protection Agency, 2004. Persistent Organic Pollutants, A
Swedish View of an International Problem. Värnamo: Fälth & Hässler.
Swedish Environmental Protection Agency, 2008. Avloppsreningsverkets förmåga att ta
hand om läkemedelsrester och andra farliga ämnen, Stockholm: Swedish
Environmental Protection Agency.
SYKE, 2008. PFOS-yhdisteiden käyttökielto voimaan 27. kesäkuuta. [Online]
[Accessed 4 October 2011].
Ternes T.A., 2004. Assessment of Technologies for the Removal of Pharmaceuticals and
Personal Care Products in Sewage and Drinking Water Facilities to Improve the
Indirect Potable Water Reuse. Project acronum POSEIDON.
Ternes, T. A. & Hirsch, R., 2000. Occurence and Behaviour of X-ray Contrast Media in
Sewage Facilities and Aquatic Environment. Environmental Science & Technology ,
Volume 34, pp. 2741-2748.
The Commission of the European Communities, 1998. COMMISSION DIRECTIVE
98/15/EC of 27 February 1998 amending Council Directive 91/271/EEC with respect to
certain requirements established in Annex I thereof. Official Journal of the European
Communities, Issue 67, pp. 29-30.
Thomas, P. M. & Foster, G. D., 2005. Tracking acidic pharmaceuticals, caffeine, and
triclosan through the wastewater treatment process. Environmental Toxicology and
Chemistry, Volume 23, pp. 25-30.
Torres, CI, Ramakrishna, S, Chiu, C-A, Nelson, KG, Westerhoff, P & Brown-
Krajmalnik, R 2011, 'Fate of Sucralose During Wastewater Treatment', Environmental
Engineering Science, Volume 28, pp. 325-331.
United States Environmental Protection Agency, 2009. Short-Chain Chlorinated
Paraffins (SCCPs) and Other Chlorinated Paraffins Action Plan. [Online]
[Accessed 27 12 2011].
United States Environmental Protection Agency, 1999. Sequencing Batch Reactors.
Washington: Office of Water.
United States Environmental Protection Agency, 2007. Method 1694: Pharmaceuticals
and Personal Care Products in Water,Soil, Sediment, and Biosolids by HPLC/MS/MS,
Washington: EPA-821-R-08-002.
Urase, T. & Kikuta, T., 2005. Separate estimation of adsorption and degradation of
pharmaceutical substances and estrogens in the activated sludge process. Water
Research, Volume 39, pp. 1289-1300.
Vesilind, A. P., 2003. Wastewater Treatment Plant Design. London: IWA Publishing.
Wick, A, Fink, G, Joss, A, Siegrist, H & Ternes, TA 2009, 'Fate of beta blockers and
psycho-active drugs in conventional wastewater treatment', Water Research, Volume
43, pp. 1060-1074.
Vieno, N., 2007. Occurence of Pharmaceuticals in Finnish Sewage Treatment Plants,
Surface Waters, and Their Elimination in Drinking Water Treatment Processes,
Tampere: Tampere University of Technology.
Vieno, N. M., Tuhkanen, T. & Kronberg, L., 2006. Analysis of neutral and basic
pharmaceuticals in sewage treatment plants and in recipient rvers using solid phase
extraction and liquid chromotography-tandem mass spectrometry detection. Journal of
Chromotography A, Volume 1134, pp. 101-111.
Vieno, N., Tuhkanen, T. & Koronberg, L., 2007. Elimination of pharmaceuticals in
sewage treatment plants in Finland. Water Research , Volume 41, pp. 1001-1012.
Wilderer, P. A., Irvine, R. L. & Goronszy, M. C., 2001. Sequenscing Batch Reactor
Technology, s.l.: IWA Scientific and Technical Report Series.
VVY, 2008. Haitallisten aineiden esiintyminen suomalaisissa yhdyskuntajätevesissä-E-
PRTR-selvityksen tulokset. Helsinki: VVY.
Zang, Y., Greissen, S.-U. & Gal, C., 2008. Carbamazepine and diclofenac: Removal in
wastewater treatment plants and occurence in water bodies. Chemosphere , Volume 73,
Zeng, L, Wang, T, Wang, P, Liu, Q, Han, S, Yuan, B, Zhu, N, Wang, Y & Jiang, G
2011. Distribution an Trophic Transfer of Short-Chain Chlorinated Paraffins in an
Aquatic Ecosystem Receiving Effluents from a Sewage Treatment Plant. Environmental
Science & Technology, Volume 45, pp. 5529-5535.
Appendix I
Appendix II
Planning and operating a pilot scale
Sequencing Batch Reactor (SBR)
Planning
When planning a SBR many things need to be taken into considerations. The most
important are listed below.
Number and operating volume of the reactors Operating cycle Pumps and tubing Timers Influent and effluent water Sludge age and sludge wasting Aeration and mixing Cleaning and maintenance of the SBRs The SBRs on weekends and holidays pH Nitrification and denitrification
Reactors and operating cycle
The number of reactors depends on the purpose of the SBRs. Many reactors enable
parallel tests to be made or the operation of multiple reactors at different conditions.
The operating volume of the reactors depends on the purpose of the SBRs and on the
equipment available. Smaller sized SBRs can be easier operated manually without many
pumps. If the operating volumes grow, larger and more complicated equipment are
needed. In the Water Laboratory at Aalto University 4 reactors are available, these work
well when the operating volume is around 10-12 liters. The four reactors are presented
in Figure 1. Extra volume in the reactors is preferable to work as buffer in case of
problems. If for example a reactor has a total volume of 30 liters it can have an
operating volume of e.g. 10-12 liters.
Figure 1 Picture of 4 reactors during aeration.
The length of the cycle depends on the planner and purpose of the reactors. A good idea
when planning the length of the cycle is to take into consideration when experiments are
made and when samples need to be taken. Cycles lasting 8 hours or less ensures that all
stages in one cycle can be observed in one normal workday. If one cycle lasts 8 hours
the SBRs operate 3 cycles per day.
The SBR cycle has five stages; fill, react, settling, draw and idle. The chosen length of
each stage is dependent on the designer. In Figure 2 an example of an 8 hour cycle is
Figure 2 Example of an 8 hour cycle.
The cycles of the reactor are controlled by aeration, pumps and mixing. These are easy
to control with a timer. It is however important to ensure that the timer is reliable and
when using multiple timers, that they work properly and in the same time. The aeration
can be arranged with an aeration stone and mixing, if needed, with a magnetic mixer.
The aeration can be strong enough to fully mix the tanks so that no additional mixing is
needed. Additional mixting is however needed if wanting to create for example anoxic
conditions. An aeration stone and a timer are presented in Figure 3.
Figure 3 Aeration stone and timer.
Pumps and tubing
The pumps and tubes should be easy to operate and maintain. Valves and the
connections between tubes should be easy to disconnect. As the tubing easily start to
accumulate biofilm and can even get clogged, the tubing should be changed regularly
(each or every second week). The tubing can even flatten and wear out. This is
important to monitor. The easiest way of doing this is to daily check the water level in
the reactors. The tubes should also not be too long or loose so that they vibrate. Too
much vibration of the tubing can affect the pumping of influent. The pumps should also
be oiled regularly, especially when the tubing is changed. Examples of pumps and
tubing are presented in Figure 4.
The accuracy of the pumps and the influence the tubes have on the yields of the pumps
are important to understand and monitor. The draw phase can be planned in a way that
the accuracy of the pumps is not that important. This can be done by attaching the
effluent tube to that height to where it is intended to remove water to. When the
accurate amount of water has been removed, more water cannot be removed even if the
pumps are still on. The feeding pumps need to be precise and their yield is important to
control so that the accurate amount of influent water is fed to the reactors.
Figure 4 Pumps and tubes.
Influent and effluent water
How much influent water is added and effluent removed, depends on the operational
parameters. More than 1/3 of the reactors' operating volume is not recommended to be
removed during a cycle. (New England Interstate Water Pollution Control Commission,
2005) A side from the organics load, when designing the amounts of water operated in
the reactors, it is important to calculate how much effluent and influent water is needed
and produced during a day and the weekends. As the reactors should operate without
maintenance a couple of days, it is important to ensure that big enough buckets and
tanks are available to hold the water. The effluent water can be led directly to the drain,
but if the sludge would escape with the effluent it can be good to catch all effluents in
separate tanks/buckets. Example of influent and effluent water tanks are presented in
As the amount of influent water is equal to the amount of effluent water, these two
should be considered together when designing the plant. The restricting parameter for
influent water is the size of the influent water tank and how often it is made. The
organics load in synthetically prepared wastewater decreases over time due to reactions
in the wastewater tank. Because of this, synthetic wastewater is good to make as often
as possible. But because the reactor should operate independently at least for a weekend
and one extra day, wastewater can be prepared every 3 or 4 days, two times a week. The
decreasing organics load should be taken into account when calculating the organics
Figure 5 Picture of influent an effluent water tanks.
One of the most important parameters when designing the SBR is the sludge
age/SRTaer. The sludge age is controlled by sludge wasting. Sludge wasting can either
be manual or automatic. A manual sludge wasting arrangement is presented in Figure 6.
Sludge can be removed only when the reactor is aerated or mixed thoroughly otherwise.
Even if the theoretical sludge wasting can be calculated as sludge removed per day or
cycle, it is not necessary to remove sludge every day. Sludge removal every other day
can be sufficient enough for longer sludge ages (> 7 days).
Figure 6 Picture of a manual sludge wasting arrangement (sludge wasted through a flexible hose).
Maintenance
The regular maintenance includes the following:
Preparing synthetic wastewater Emptying effluent water tanks Removing sludge Keeping the reactor walls clean from sludge Observing the yield of pumps Checking the tubes and change them when necessary Analyzing and following how the reactors are operating
Analyses
The most important analyses to make on regular bases are MLSS and the organics
analyses. The MLSS in the reactors tells how the sludge is adapting to the conditions in
the reactors. If the MLSS sinks drastically after the start of the reactors, this can mean
that the organics load is not sufficient. The organics load tells if the reactors are
removing organics as planned. The pH is also important to monitor on an almost daily
bases as this tells if the dose of chemicals that adds alkalinity is correct. Nitrification
can also lower the pH in the process. Dissolved oxygen can also be measured regularly.
The amount of oxygen in the reactors can be controlled. If however mixing with only
aeration is wanted then the aeration needs to be strong all the time. Other analyses can
be made depending on the purpose of the reactors.
Possible problems
Denitrification and surface sludge
Sudden rises of organics loads can lead to problems in the reactors. If the tubing has
stayed unchanged for long, they can have formed biofilm or other accumulation that
then decrease the organics load that reach the reactors. When the tubing is then changed,
this can lead to a larger organics load than the reactors are used to. In case the SBR is
designed only to nitrify and denitrification starts and the reaction has a long time to take
place, surface sludge can became a problem. A risk is that if the surface sludge forms
before the draw stage of the cycle, the sludge is removed with the effluent water. As a
conclusion, the tubing should be changed regularly to keep the actually fed organics
load relatively constant. The react stage should also be long enough to ensure that
organics are removed and the settling stage not so long that the anoxic conditions that
make denitrification possible can form. The settling stage should however be long
enough to ensure proper settling to occur. A picture of surface sludge is presented in
Figure 7 Picture of surface sludge in a SBR (most likely caused by denitrification).
Settling of sludge
When choosing the operating parameters such as MLSS concentration and organics
loads for the SBRs, the settling of sludge is good to take into consideration. If the
settling is not good and the loads and MLSS concentrations are high, the settling of
sludge can be a problem. Also excessive foaming in the reactors can be a problem and
anti-foaming agents can be used.
General tips
It is preferable to regularly check the reactors and observe the different stages of the
cycles. Always when some change is made the following cycles are good to observe.
Surprises and unexpected reactions to small changes can occur.
Holidays and unexpected sickness are good to be prepared for. If the primary person
who is responsible for the SBRs is sick, then someone else should be appointed to step
in to ensure that the operation continues as usual. This means that more than one person
needs to be familiar with the operation.
References
New England Interstate Water Pollution Control Commission, 2005. Sequencing Batch
Reactor Desing and Operational Considerations, Massachusetts
Source: http://civil.aalto.fi/fi/midcom-serveattachmentguid-1e469908479103a699011e4bdf5f787f9d1674b674b/ahlgren2012.pdf
E,RISKINGDEATHM LIFE, GIVING LIFE, RISKING DEATH MATERNAL MORTALITY IN BURKINA FASO More than 2,000 women die in Burkina Faso every year from complications of pregnancy and childbirth. Most of these deaths could be prevented. Some women die because they cannot reach a health facility capable of treating them, or because they arrive too late. Many lose their livesbecause their relatives cannot pay the fees demanded by medical
Nutrient Profile of Nutritional Support for CNS Disorders Copyright © January, 2001 Truehope Nutritional Support Ltd. A Non-Profit Company Revised August, 2006 Table of Contents 1. Nutrient Insufficiency Theory.2 2. Nutrient Profile .3 3. Possible Interfering Factors.28 4. Conclusion .32 Preface There are many good reasons to suspect that nutrition, or the lack of it, may be the root cause of many of our chronic illnesses, including disorders of the central nervous system. Small population pockets in a few places around the world such as the Hunza population in Kashmir, the Georgians from the southern Soviet Union and the mountain village of Vilcabamba in Ecuador are reported to live much longer and remain more vigorous in old age than in most modern societies.1 The Japanese island of Okinawa also shares a similar reputation, and like these other populations enjoys life with little or no chronic or mental illness. One has to ask why such a phenomenon occurs. The only common factor shared by these diverse populations seems to be the incredible macro and micronutrient density of their foods and water coupled with rich soils, which do not suffer from depletion. These populations ingest many times the macro and microelement levels ingested by the average North American. Around the world, soil depletion of nutrients is at a very high level. The statistics from the 1992 Earth Summit Report indicate that 85% of the minerals present in North American topsoils 100 years ago are no longer present. This correlates with the findings of Anne-Marie Mayer, PhD who reported that the general decline of nutrients in 40 foods examined over a 50-year period of analysis in the UK reached a peak of an 81% decrease for a single element.2 This decrease in nutrient density in our foods could easily have been predicted; as for hundreds of years the principle elements replenished in farm soils have been only nitrogen, phosphorus and potassium. The over-processing of foods has also, no doubt, contributed to the problem of low nutrient intake. White flour, for instance, contains only 7% of the trace elements found in whole-wheat flour.3 Another factor which figures into this equation is the load put on body resources to metabolize and eliminate environmental contaminants and pollutants. Various chemicals including herbicide and pesticide exposure, not to mention the numerous airborne toxins that our bodies are constantly exposed to, put an overload on body metabolic processes and resources to counter such exposure.