Ck1-lab.de
240 European Journal of Cell Biology 79, 240 ± 251 (2000, April) ´ Urban & Fischer Verlag ´ Jena
Interaction of casein kinase 1 delta (CK1d) with post-
Golgi structures, microtubules and the spindle
Lars Behrend, Martin Stöter, Marion Kurth, Gabriel Rutter, Jochen Heukeshoven, Wolfgang Deppert,
Uwe Knippschild1)Heinrich-Pette-Institut für Experimentelle Virologie und Immunologie an der Universität Hamburg, Hamburg/Germany
Received August 21, 1999
Received in revised version January 11, 2000
Accepted January 11, 2000
Casein kinase 1 delta ± trans Golgi network ± mitosis ±
isoforms differ in tissue distribution and subcellular localisa-
microtubules ± phosphorylation
tion, CK1 protein kinases share several characteristics. All
CK1 family members exhibit Ser/Thr-specific protein kinase
activity, and some isoforms from yeast can also phosphorylate
Members of the casein kinase 1 family of serine/threonine
tyrosine (Hoekstra et al., 1994). They exclusively use ATP as a
kinases are highly conserved from yeast to mammals and seem
phosphate donor, are generally cofactor-independent, and
to play an important role in vesicular trafficking, DNA repair,
depend on N-terminal acidic and/or phosphorylated amino
cell cycle progression and cytokinesis. We here report that in
acids for substrate recognition (Roach, 1990; Tuazon and
interphase cells of various mammalian species casein kinase 1
Traugh, 1991). CK1 protein kinases also contain a putative
delta (CK1d) specifically interacts with the trans Golgi net-
nuclear localisation signal (Rowles et al., 1991; Tuazon and
work and cytoplasmic, granular particles that associate with
Traugh, 1991; Zhai et al., 1995) and possess a kinesin homology
microtubules. Furthermore, at mitosis CK1d is recruited to the
domain (Roof et al., 1992; Xu et al., 1995), which in kinesins is
spindle apparatus and the centrosomes in cells, which have
necessary for interactions with microtubules. All CK1 isoforms
been exposed to DNA-damaging agents like etoposide or g-
exhibit a high degree of sequence conservation within their
irradiation. In addition, determination of the affinity of CK1d
kinase domains, but differ significantly in length and primary
to different tubulin isoforms in immunoprecipitation-Western
structure of their C-terminal non-catalytic domains. These
analysis revealed a dramatically enhanced complex formation
variable C-terminal domains are responsible for substrate
between CK1d and tubulins from mitotic extracts after
specificity of the different isoforms (Cegielska et al., 1998;
introducing DNA damage. The high affinity of CK1d to the
Graves and Roach, 1995) and are involved in the regulation of
spindle apparatus in DNA-damaged cells and its ability to
interaction with other proteins, and/or subcellular structures.
phosphorylate several microtubule-associated proteins points
Phosphorylation (Cegielska et al., 1998), and perhaps dimer-
to a regulatory role of CK1d at mitosis.
ization (in the case of CK1d) (Longenecker et al., 1998), are
additional mechanisms that regulate CK1 activity, specificity,
and subcellular localisation.
The wide spectrum of substrates suggests that the CK1
kinase family might be involved in many different cellular
The casein kinase 1 (CK1) family of protein kinases is common
processes. However, so far only a few examples link CK1
to all eukaryotic cells. At least fifteen sequence-distinct
activity to specific functions in vivo (Gross and Anderson,
mammalian isoforms have been characterised: isoforms a, b,
1998; Peters et al., 1999; Sakanaka et al., 1999).
g1 ± 3, d and e (Fish et al., 1995; Graves et al., 1993; Gross and
The function of CK1-related kinases has been best analysed
Anderson, 1998; Rowles et al., 1991; Zhai et al., 1995) and
in yeast; four different CK1 homologues have been identified
their various splice variants (Zhai et al., 1995). Although the
(Christenson et al., 1997). Some of these CK1 homologues
participate in the regulation of membrane transport processes
(Murakami et al., 1999; Panek et al., 1997), in cell morphogen-
Dr. Uwe Knippschild, Heinrich-Pette-Institut für Experimentalle
Virologie und Immunologie an der Universität Hamburg, Martini-
esis (Robinson et al., 1993) and in DNA repair pathways
straûe 52, D-20251 Hamburg/Germany, e-mail:
[email protected]
(Dhillon and Hoekstra, 1994). For example, in S. cerevisiae the
hamburg.de, Fax: 494048051117.
hrr25 gene encodes a CK1-related kinase, which is an essential
Interaction of CK1d with post-Golgi strucures, microtubules and the spindle apparatus 241
component of DNA repair processes. Interestingly, hrr25
Bristol, GB), cathepsin D (kindly provided by Annette Hille-Rehfeld,
mutants can be complemented by the mammalian CK1d and
Göttingen, Germany), K58 (Sigma), rab5, rab9, a-adaptin, g-adaptin,
e isoforms, but not by the a isoform (Hoekstra et al., 1994),
and dynamin II (Santa Cruz, California, USA), respectively.
suggesting that those two isoforms might have similar func-
tions in mammals. CK1 d/e have also been implicated in the
regulation of the tumour suppressor p53 (Knippschild et al.,
Cells used for the immunofluorescence microscopy were grown on
1997). The induction of CK1d by p53 in stress situations and its
coverslips or, in the case of mitotic shake off, cells were prepared by
ability to phosphorylate p53, suggest that it may play a central
cytospin in a cytospin 3 centrifuge (Shandon) at 1000 rpm for 10
minutes. Cells were washed twice in PEM (0.1 M PIPES, pH 6.9; 5 mM
role in mediating the downstream effects of p53 on cell growth
and genome integrity (Knippschild et al., 1996; Knippschild
2; 1 mM EGTA) and fixed in 3% paraformaldehyde in PEM for 10
minutes at 378C. In some cases, cells were treated with the micro-
et al., 1997).
tubules-effecting drugs nocodazole (5 mM) or vinblastine (2 mM) in
Here we report that in interphase cells CK1d is found in
medium and fixed as above. Fixed cells were permeabilised in PBS
association with the trans Golgi network (TGN), and in
containing 0.3% Triton X-100 at 378C for 3 minutes. Where indicated,
addition with cytoplasmic granular particles, which decorate
cells were preextracted up to 20 minutes either with KM buffer (10 mM
microtubules. During mitosis CK1d is evenly distributed
2-[N-morpholino] ethane sulphonic acid [MES], pH 6.8, 10 mM sodium
throughout the cytoplasm. However, upon genotoxic stress
chloride, 1.5 mM magnesium chloride, 5 mM dithiothreithol (DTT),
induced by etoposide or g-irradiation, CK1d specifically
1 mM EGTA, 50 mM leupeptin, 30 mg/ml aprotinin) supplemented with
1% NP40 or up to 10 minutes in PEM supplemented with 3% Triton X-
interacts with the mitotic spindle apparatus. Immunoprecipi-
100. Where indicated, cells were treated with the membrane transport-
tation-Western blot analyses showed that CK1d binds to all
affecting drug brefeldin A (BFA, 5 mg/ml in medium) and fixed as
tubulin isoforms and that its affinity to tubulin isoforms
described above. Fixed cells were washed in PBS at room temperature
changes during the cell cycle and upon DNA damage. Our
and blocked in PBS containing 0.2% gelatine for 1 hour followed by an
results therefore suggest that CK1d participates in regulatory
incubation with primary antibodies. For multiple staining, primary
processes at the onset of mitosis.
antibodies were incubated sequentially for 45 minutes each. Secondary
antibodies were bound for 30 minutes using Cy2-, Cy3-, FITC- or Texas-
Red-labelled IgG (DIANOVA, Germany), respectively. The above
Materials and methods
incubation steps were each finished by washing in PBS (4 ± 8 ). DNA
was visualised by DAPI staining (15 minutes; 0.1 mg/ml). Epifluores-
cence microscopy was performed using a Leica 63 /1.4 or 40 /1.0 oil
immersion objective on a Leica DM-IRS microscope equipped with a
Primary mouse embryo fibroblast (MEF) cultures were prepared from
confocal imaging system (TCS NT-Version) or with a Leica DM-R
mechanically dispersed 17-day-old Balb/c mouse embryos by digestion
microscope using a high-resolution digital camera system (Diagnostic
with 0.2% collagenase in phosphate-buffered saline (PBS). Primary
Instruments, Intas, Germany).
MEF cultures, C57MG cells (Vaidya et al., 1978), Balb/c 3T3 cells
(Aaronson and Todaro, 1968), and F111 cells (Freeman et al., 1975)
Preparation of cytoskeletons and
were grown in Dulbeccos modified Eagles medium (DMEM)
immunoelectron microscopic detection of CK1d
supplemented with 10% FCS at 378C in a humidified 5% CO2
For electron microscopy, glass coverslip cultures of MEFs were washed
and stabilised in PEM supplemented with 10% DMSO for 5 minutes at
room temperature. Cells were then extracted at room temperature in
Cell cycle enrichment
the same buffer supplemented with 0.5% Triton X-100 and 1%
F111 cells, p53 -/- fibroblasts or MEFs were seeded on 10-cm plates. For
aprotinin (Bayer Leverkusen, Germany). The cytoskeleton was fixed in
arrest in G1/S phase, cells were treated with aphidicolin (1 mg/ml) and
PEM buffer containing 0.5% glutaraldehyde and 4% paraformalde-
thymidine (2.5 mM) for 17 hours, then released for 8 hours and once
hyde, washed and then blocked with 1% bovine serum albumin for 20
again treated with aphidicolin and thymidine. For the enrichment of
minutes. CK1d was detected by incubation with a 1:200 dilution of the
cells in G2/M phase, cells were harvested 8 to 12 hours after the second
polyclonal rabbit antiserum NC10 for 30 minutes at room temperature.
aphidicolin/thymidine block. In some cases, cells were g-irradiated (1 to
Subcellular localisation of CK1d detected by the NC10 serum was
5 gray) or treated with etoposide (0.2 to 1 mM) 30 minutes after release
visualised by an additional incubation with 9 nm gold particles
from G1/S arrest and then harvested at G2/M. The mitotic cells were
conjugated to protein A for 30 minutes at room temperature. After
either used for immunofluorescence microscopy, immunoprecipitation,
repeated washing, the extracted cells were postfixed with 2.5%
or Western blotting.
glutaraldehyde in buffer for 10 minutes and further stained with 2%
aqueous solution of uranyl acetate for 30 minutes at 48C. The extracted
Antibodies and production of antibodies
cells were dehydrated through a graded serious of cold ethanol and
The peptide CGDMASLRLHAARQGARC (rat CK1d-specific C-
dried from tetramethylsilane. The dried cytoskeletons were shadowed
terminal sequence) was coupled to KLH for the first, to BSA for the
with carbon at a 908 angle in vacuum (10ÿ7 mbar), floated on
second, and to ovalbumin for the third immunisation. For the
hydrofluoric acid, washed in distilled water, and mounted on copper
immunisation of rabbits, the coupled peptide emulsified in an equal
grids without a supporting film. Micrographs were taken in a Philips
volume of complete (first immunisation) or incomplete (subsequent
CM 120 transmission microscope.
immunisations) Freunds adjuvant (Gibco Laboratories) was used for
subsequent subcutaneous injections in rabbits. Test bleed of each
Lysis, immunoprecipitation, SDS-PAGE and
animal was taken 10 days after each boost, and after three to four boosts
Western immunoblotting
the animals were bleeded out.
Cells were washed in ice-cold PBS and extracted for 30 minutes with
This work was initiated by using the CK1d-specific monoclonal
lysis buffer (TK-buffer) containing 50 mM Tris-HCl (pH 9.0), 25 mM
antibody 128A, which was kindly provided by ICOS Corporation
KCl, 10% glycerol, 5 mM DTT, 1 mM EDTA, 1 mM EGTA, 50 mM
(Washington, USA). Additionally, polyclonal antibodies against a/b-
leupeptin, 30 mg/ml aprotinin, and 1% empigen. Extracts were cleared
tubulin (kindly provided by Gabriel Rutter, Hamburg, Germany), g-
by centrifugation for 45 minutes at 12000g at 48C, diluted 1: 4 with a
tubulin (Sigma, Germany) and a monoclonal anti-b-tubulin antibody
buffer containing 50 mM Tris-HCl, pH 8.0, 150 mM NaCl, 10%
(Boehringer, Germany) were used. As markers for membrane organ-
glycerol, 0.5% NP40, 5 mM DTT, 1 mM EDTA, 1 mM EGTA, 50 mM
elles we used antibodies against TGN38 (a gift from George Banting,
leupeptin, and 30 mg/ml aprotinin, then subjected to immunoprecipita-
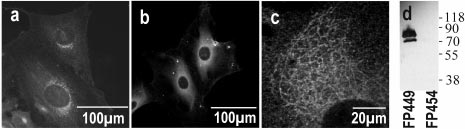
242 L. Behrend, G. Rutter et al.
tion using antibodies specific for either tubulins or CK1d and incubated
polyacrylamide gels, blotted onto a nitrocellulose membrane (Hybond)
with 50 ml of settled protein A-Sepharose beads on a rotating shaker for
and isolated. Membrane fragments containing phosphate-labelled
2 hours at 48C. The immunoprecipitates were washed five times with
proteins were incubated in 100 mM acetic acid with 5% polyvinylpyr-
PBS containing 1% NP40, three times with PBS, and once with 0.1
rolidone (PVP-360, Sigma, Germany) at 378C for 45 minutes,
PBS. The immunocomplexes were denatured in sodium dodecyl sulfate
extensively washed with water and 50 mM ammonium bicarbonate
(SDS) sample buffer, separated on SDS-polyacrylamide gels, and
buffer, digested with trypsin and oxidized with performic acid for 1 h.
transferred to a nitrocellulose membrane (Hybond C super, Amer-
Phosphopeptides of either tubulin, stathmin or MAP4640±1125 were analysed
sham). The membranes were probed with CK1d or tubulin specific
on cellulose thin layer plates by electrophoresis at pH 1.9 followed by
antibodies. Immunocomplexes were detected using either horse radish
ascending chromatography in a buffer containing either n-butanol/
peroxidase-conjugated anti-rabbit IgG (SAPU, Scotland, GB) or anti-
pyridine/acetic acid/H2O, 15:10:3:12 (vol/vol) (Knippschild et al., 1997),
mouse peroxidase-conjugated IgG, followed by chemiluminescence
or isobutyric acid/pyrimidine/acetic acid/n-butanol/H2O, 65:5:3:2:29.
detection (ECL, Amersham, Germany and Pierce, USA).
Expression and purification of glutathione S-
transferase fusion proteins
In this study two glutathione S-transferase (GST) fusion proteins were
used: FP449 contains the full sequence of rat CK1d fused to the C-
Subcellular localisation of CK1d in interphase
terminus of GST in the vector pGEX-2T (Pharmacia, Sweden),
whereas FP455 comprises the full sequence of human CK1e (both
We first examined the subcellular localisation of CK1d in Balb/
plasmids were kindly provided by David Meek, Scotland, UK). The
c 3T3 and F111 cells using CK1d specific antibodies for the
construction, expression and purification of these proteins are de-
detection of CK1d by indirect immunofluorescence micros-
scribed in detail elsewhere (Knippschild et al., 1997).
copy. Staining of CK1d in Balb/c 3T3 cells with the monoclonal
antibody 128A (Fig. 1a) or staining of F111 cells with the
In vitro kinase assays
polyclonal serum NC10 (Fig. 1b) revealed a pronounced
In vitro kinase assays were carried out as previously described
cytoplasmic distribution of CK1d, with a predominant peri-
(Knippschild et al., 1996) using either bovine tubulin (ICN, California,
nuclear localisation (Western blot analysis revealed that NC10
USA), the GST-stathmin fusion protein (kindly provided by Irene
specifically recognises CK1d and its hyperphosphorylated
Dornreiter and Silke Dehde, Hamburg, Germany), tau or the
MAP4640±1125 fragment (both proteins were a gift from Eva-Maria
form, see Fig. 1d). Additionally, we found a weaker staining of
Mandelkow, Hamburg, Germany) as substrate. In each case, the protein
CK1d in the peripheral cytoplasm, which seems to be
was resuspended in a total volume of 20 ml containing 25 mM Tris-HCl
organised in linear arrays (Fig. 1a,b). Similar results were
(pH 7.5), 10 mM MgCl
obtained in all cell lines analysed (C57MG, MCF7, swiss 3T3,
2, 0.1 mM EDTA and 100 mM [g-32P]ATP
(specific activity 5 Ci/mmol). The kinase reactions were started by
data not shown).
adding the C-terminal truncated CK1d (Calbiochem, Germany) or the
Enlarged laser scan images of MEFs showed that the
full length GST-CK1d fusion protein FP449 and incubated for 30
perinuclear structure stained by CK1d-specific antibodies
minutes at 308C. The reactions were stopped by adding 5 ml of
appeared as a tubular and cisternal network (Fig. 1c), which
concentrated SDS sample buffer as described elsewhere (Knippschild
is sensitive to detergent treatment (data not shown).
et al., 1997). The phosphorylated proteins were separated by SDS-
polyacrylamide gel electrophoresis and detected by autoradiography.
CK1d localises to the TGN and clathrin-coated
Phosphopeptide analysis
membranes of the TGN region
In vitro phosphorylated tubulin, stathmin, tau and MAP4640±1125 were
CK1d seems to be associated with a membrane network. Since
analysed by two dimensional phosphopeptide analysis using a standard
the fungal secondary metabolite brefeldin A (BFA) induces
protocol as described earlier (Knippschild et al., 1995). In vitro
fundamental changes in morphology and dynamics of several
phosphorylated proteins were separated by SDS-PAGE on 10%
membranous organelles of the secretory pathway (Lippincott-
Fig. 1. Subcellular localisation of CK1d in interphase cells. To
enlarged laser scan image of the perinuclear, reticular CK1d-positive
determine the subcellular localisation of CK1d in interphase cells,
structures in MEFs is shown in c. Bars as indicated. Western Blot
Balb/c 3T3, F111 cells and MEFs, respectively were grown on cover-
analyses were performed to confirm the specificity of the CK1d-specific
slips, then fixed and stained as described in Material and methods. Balb/
polyclonal serum NC10 (d). The GST-CK1d fusion protein FP449 was
c 3T3 cells were stained with the monoclonal anti-CK1d specific
detected from bacterial extracts, whereas the GST-CK1e fusion protein
antibody 128A (lane a) (Icos Corporation, Washington, USA), while
FP455 was not recognised by NC10 (Fig. 1d) (both plasmids were
F111 cells were stained with the CK1d-specific polyclonal serum NC10
kindly provided by David Meek, Scotland, UK).
(b) followed by labelling with a Texas Red-conjugated IgG each. An
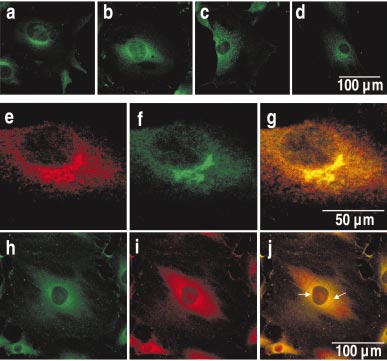
Interaction of CK1d with post-Golgi strucures, microtubules and the spindle apparatus 243
Schwartz et al., 1991) we analysed the effects of BFA on the
et al., 1991; Strous et al., 1993). We therefore attempted to co-
morphology of CK1d-positive, perinuclear structures. F111
localize CK1d with proteins residing in distinct organelles of
cells were treated with 5 mg/ml BFA, fixed at different time
the secretory pathway. However, double staining of CK1d with
points (30 minutes, 60 minutes, and 5 hours after addition of
the Golgi-specific protein K58 did not result in co-localisation
BFA) and stained for CK1d (Fig. 2b ± d). Compared to
of both proteins in the laser scan microscopy (data not shown).
untreated cells (Fig. 2a), the distribution of CK1d changed
Furthermore, we analysed the distribution of TGN38, an
from the typical perinuclear, reticular-like staining to a more
integral membrane protein of the TGN (Fig. 2e), and CK1d
vesicular-like staining of CK1d up to 60 minutes after
(Fig. 2f) in F111 cells by laser scan microscopy. The merged
treatment with BFA, which extended more to the periphery
image of CK1d and TGN38 clearly demonstrates a co-
of the cytoplasm (Fig. 2b,c). However, after long-term treat-
localisation of both proteins in the perinuclear region (Fig. 2g).
ment (5 hours) the CK1d-positive structures nearly completely
To elucidate the identity of the less detergent sensitive
condensed into a stable structure in the perinuclear region
staining of CK1d in the periphery of the cytoplasm, we
(Fig. 2d). The observed BFA effects were reversible, as the
examined the co-localisation of CK1d with vesicular and
original perinuclear reticular-like staining of CK1d was largely
endocytotic marker proteins. Double staining of CK1d either
re-established already 30 minutes after BFA release (data not
with early (rab5) or late endosomes (rab9), lysosomes
shown). The pronounced effect of BFA on the perinuclear
(cathepsin D) (data not shown), or clathrin-coated vesicular
reticular structures stained by CK1d-specific antibodies sug-
structures (g-adaptin and a-adaptin) (Fig. 2h ± j, data not
gests an association of CK1d with membrane structures of the
shown) were performed and analysed by laser scan micros-
secretory pathway and is similar to the behaviour of post-Golgi
copy. Furthermore, we proved whether CK1d co-localises with
organelles (Ladinsky and Howell, 1992; Lippincott-Schwartz
dynamin II known to be involved in endocytotic processes and
Fig. 2. CK1d co-localises with the trans Golgi network (TGN) and
network (TGN) (Fig. 2e ± g). F111 cells were fixed, permeabilised and
AP1-positive membrane regions. To analyse alterations in the sub-
then incubated with antibodies to TGN38 (e) followed by labelling with
cellular localisation of CK1d upon BFA treatment F111 cells were
secondary Cy3-conjugated antibodies and to CK1d (f) followed by
incubated with BFA (5 mg/ml) either for 30 minutes (b), 60 minutes (c),
incubation with FITC-labelled antibodies. Overlays are shown in
or 5 hours (d) at 378C. Untreated (a) and treated cells (b ± d) were
panel g. Bar, 50 mm. Double staining of F111 cells for CK1d and g-
fixed, permeabilised and then incubated with antibodies specific for
adaptin are presented in panels h ± j. h: g-adaptin, i: CK1d, j: merged
CK1d followed by FITC anti-rabbit IgG. Bar, 100 mm. Laser scan
image of CK1d and g-adaptin. Regions of co-localisation are indicated
microscopy revealed a co-localisation of CK1d with the trans Golgi
by arrows. Bars as indicated.
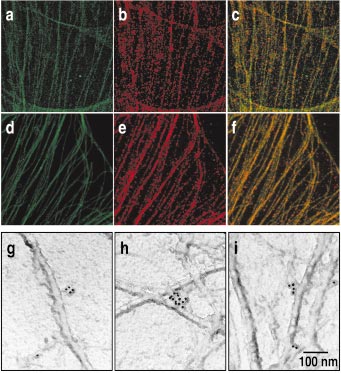
244 L. Behrend, G. Rutter et al.
to interact with clathrin-coated vesicles and microtubules
shown). Therefore, we asked whether the CK1d-stained
(data not shown). However, only in the case of g-adaptin
particles were associated with the microtubule network. For
(Fig. 2h), a clathrin-binding protein that resides to post-Golgi
this purpose, MEFs were double stained for a/b-tubulin
structures (Wong and Brodsky, 1992), we did observe a partial
(Fig. 3a,d) and CK1d (Fig. 3b,e) and analysed by laser scan
co-staining with CK1d (Fig. 2i) in the perinuclear Golgi region
immunofluorescence microscopy. In such regions, where single
of F111 cells by epifluorescence microscopy (see arrows).
microtubules could be distinguished, an association of the
These results indicate that CK1d localises at the TGN and
small, CK1d-positive granular particles with microtubules was
clathrin-coated areas of the TGN region.
observed (Fig. 3c,f). The merged images of a/b-tubulin and
CK1d staining (Fig. 3c) clearly revealed that CK1d-positive
CK1d-positive granular particles are
granular particles decorate the microtubules as spots. Inter-
associated with microtubules
estingly, after detergent treatment, (Fig. 3d ± f) CK1d re-
We did not observe a co-localisation of CK1d-positive granular
mained bound to microtubules indicating that it is tightly
particles with endosomal/lysosomal or vesicular marker pro-
bound, directly or mediated by an unknown linker, to the
teins in the cell periphery. Since those particles were aligned in
linear arrays we assume an association with filamentous
To support a close association of CK1d with microtubules we
structures (Fig. 1a). To clarify this observation, MEFs were
performed immunoelectron microscopy with detergent-ex-
double stained with CK1d-specific antibodies and either
tracted cytoskeletons of MEFs (see Materials and methods).
antibodies against vimentin (intermediate filaments) or actin
Therefore, F111 cells were extracted and labelled with the
(actin filaments). However, no association between CK1d with
polyclonal CK1d-specific antiserum NC10 and protein A-gold.
any of these two filament systems could be observed (data not
The gold particles labelled clusters that are attached to
Fig. 3. CK1d-positive granular particles are tightly associated with
f. Immunoelectron microscopic analysis of cyctoskeleton preparations
microtubules in interphase cells. Untreated MEFs (a ± c) or MEFs
of MEFs using the CK1d-specific polyclonal serum NC10 and second-
treated with detergent for 20 minutes (d ± f) were fixed, permeabilised
ary immunogold-labelled antibodies are shown in g, h, i. Micrographs
and incubated with antibodies specific to a/b-tubulin (a, d) and to CK1d
taken in a Philips CM 120 transmission microscope underline that the
(b, e), followed by incubation with Texas Red- and FITC-labelled
9-nm immunogold particles trace CK1d-containing clusters attaching
secondary antibodies. The merged laser scan images are shown in c and
microtubules. Magnification 110000. Bars as indicated.
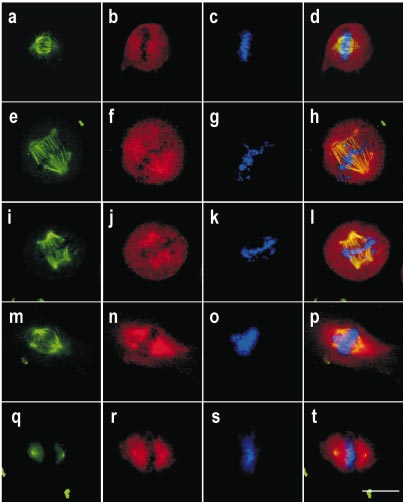
Interaction of CK1d with post-Golgi strucures, microtubules and the spindle apparatus 245
filamentous structures. These filaments with a diameter of 22 ±
and Nelson, 1996). The assembly of the bipolar mitotic spindles
24 nm were identified as microtubules (Fig. 3g,h, i).
involves a complex rebuilding of microtubules (Zhai et al.,
1996). As CK1d is associated with the microtubule network in
Subcellular localisation of CK1d in mitotic cells
interphase cells, we asked whether the association of CK1d
In interphase cells, microtubules play an important role in
with tubulin is maintained during mitosis. F111 cells enriched
vesicle transport, cell migration and in maintaining cellular
in mitotic stages (see Material and methods) were subjected to
architecture. During mitosis, tubulin builds up the mitotic
cytospin and analysed for the association of CK1d with the
spindle that is involved in chromosome segregation (Drubin
spindle apparatus by immunofluorescence (Fig. 4a ± d). Chro-
Fig. 4. CK1d binds to the spindle apparatus if segregation failures
fixed, permeabilised and incubated with anti-a/b-tubulin (a, e, i, m) or
occur. F111 cells (a ± d) were released from G1/S-phase block as
g-tubulin antibodies (q) and with antibodies against CK1d (b, f, j, n, r)
described in Materials and methods. Alternatively, F111 cells (e ± l,
followed by incubation with secondary Cy3- and FITC-labelled
q ± t) were treated with etoposide (e ± h) or g-irradiated (i ± l, q ± t) as
antibodies. Chromosomes were visualised by DAPI staining (c, g, k,
described in Material and methods. Images of an untreated MEF cell
o, s). Merged images of all three stainings are presented in d, h, l, p, and
with an apparent centrosome defect are presented in m ± p. Cells were
t. Bar, 10 mm.
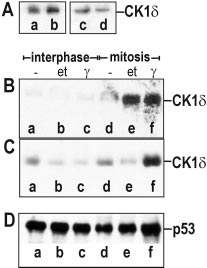
246 L. Behrend, G. Rutter et al.
mosomes were concomitantly detected by staining with DAPI.
CK1d showed an even distribution throughout the whole cell
(Fig. 4b), and was slightly increased around the chromosomes.
Although the merged image of tubulin and CK1d staining does
not point to a co-localisation of CK1d with the mitotic spindle,
we cannot exclude a weak association of CK1d which is
undetectable by immunofluorescence techniques.
There is evidence that CK1d is activated upon genotoxic
stress in a p53-dependent manner (Knippschild et al., 1997),
thereby mediating signal integration onto p53. This leads to a
modified p53 response and activation of cell cycle checkpoints
(Meek, 1998; Schwartz et al., 1997; Schwartz and Rotter, 1998).
To get hints for a possible involvement of CK1d in mitotic
checkpoint control, G1/S-arrested F111 cells, either g-irradi-
ated or treated with etoposide shortly after their release from
the arrest, were analysed during mitosis to detect changes in
the affinity of CK1d to the mitotic spindle. Interestingly, in
both, etoposide-treated (Fig. 4e ± h) and g-irradiated cells
(Fig. 4i ± l) we found a large amount of CK1d co-localised
with the mitotic spindle (Fig. 4h,l). Thereby, CK1d was not
evenly distributed but particularly aligned to the spindle. The
spindle staining of CK1d in g-irradiated cells was mostly
accompanied by a microscopically detectable defect in the
mitotic spindle and frequent abnormalities in the chromatin
structure characterised by a low condensation status (Fig. 4k).
However, in etoposide-treated cells we always found spindle
failure, the formation of multiple centrosomes and damage of
Fig. 5. Detection of complexes between CK1d and tubulin during the
the chromatin structure (Fig. 4g).
cell cycle and upon genotoxic stress. A: a/b-tubulin (a, b), or g-tubulin
Without inducing DNA damage we observed a strong co-
(c, d) were immunoprecipitated from lysates of asynchronously
staining of CK1d with the mitotic spindle only in the case
growing (a, c) or mitotic F111 cells (b, d). The immunoprecipitates
were separated by SDS-PAGE, blotted onto nitrocellulose and probed
presented in Fig. 4m ± p. In these MEFs an apparent defect in
for CK1d (see Material and methods). B, C, D: Immunoprecipitation-
centrosome division is indicated by the presence of three
Western analysis of a/b-tubulin and CK1d are shown in B, those of g-
instead of two spindle poles. Such centrosome division failure
tubulin and CK1d in C, whereas immunoprecipitation-Western analysis
increases the incidence of multipolar mitosis, which leads to
of p53 is presented in D. Cell lysates were obtained from cells growing
chromosome segregation abnormalities (Lingle et al., 1998).
under various conditions; a: asynchronously growing cells, b: asynchro-
This could also be seen in the DAPI staining of the untreated
nously growing cells treated with 0.2 mM etoposide (et), c: g-irradiated
MEF where multipolarity led to an inadequate alignment of
(g) (1 gray) asynchronously growing cells, d: mitotic shake-off cells, e:
the metaphase chromosomes (Fig. 4o). The dramatic effect of
mitotic shake-off cells treated with 0.2 mM etoposide (et), f: g-irradiated
such centrosome division defects underlines the important role
(g) (1 gray) mitotic shake-off cells.
of the centrosomes as part of the chromosome segregation
machinery. To obtain information whether CK1d also interacts
CK1d co-precipitated with a/b-tubulin slightly increased in
with the centrosomes in addition to the spindle, g-irradiated
mitotic cells (Fig. 5A, lane b) as compared to asynchronously
cells were co-stained for CK1d and g-tubulin, an important
growing cells (Fig. 5A, lane a). In contrast, the amount of
component of the centrosomes (Moritz et al., 1995) (Fig. 4q ±
CK1d co-precipitated with g-tubulin remained unchanged
t). CK1d was found to be located throughout the whole cell
(Fig. 5A, lanes c and d) irrespectively of the synchronisation.
with a pronounced staining at the centrosome region (Fig. 4r).
By implementation of the reciprocal immunoprecipitation we
The merged image of g-tubulin and CK1d (Fig. 4t) demon-
detected all indicated tubulin isoforms in CK1d immunopre-
strates that a fraction of CK1d is indeed associated with the
cipitates (data not shown).
centrosomes during mitosis after induction by DNA damage.
Furthermore, we determined the effect of genotoxic stress
on the binding of CK1d to a/b- or g-tubulin. Fig. 5B shows an
The affinity of CK1d to tubulin changes during
immunoprecipitation-Western analysis of CK1d in asynchro-
the cell cycle and upon genotoxic stress
nously growing cells immunoprecipitated with a/b-tubulin
Our immunofluorescence data show that CK1d is associated
in either untreated, etoposide-treated or g-irradiated cells
with microtubules in interphase cells, and with the spindle appa-
(Fig. 5B, lanes a, b, c), or in mitotic cells treated respectively
ratus and the centrosomes in mitotic cells after DNA damage.
(Fig. 5B, lanes d, e, f). Whereas g-irradiation and etoposide
Cellular stress situations lead to alterations in the activity of CK1d
treatment of asynchronously growing cells had only little effect
(Knippschild et al., 1996; Knippschild et al., 1997). These altera-
on the association of CK1d with a/b-tubulin (Fig. 5B, lanes b,
tions might influence its affinity to tubulin. Therefore we asked,
c), these treatments strongly stimulated the binding of CK1d to
whether CK1d co-immunoprecipitates with different tubulin
tubulin isolated from aphidicolin/thymidine-synchronised mi-
isoforms during the cell cycle and after genotoxic stress.
totic cells. This dramatic increase in the amount of co-
Indeed, we found CK1d in complex with a/b- and g-tubulin
precipitated CK1d can be seen after DNA damage induced
in asynchronously growing and in mitotic F111 cells in im-
by either etoposide (Fig. 5B, lane e) or g-irradiation (Fig. 5B,
munoprecipitation-Western analysis (Fig. 5A). The amounts of
lane f) 30 minutes after release from G1/S.
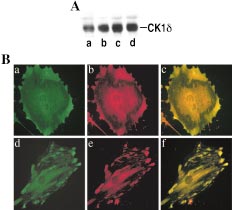
Interaction of CK1d with post-Golgi strucures, microtubules and the spindle apparatus 247
Binding of CK1d to g-tubulin (Fig. 5C) showed some
the amounts of co-immunoprecipitated CK1d compared to
differences compared to those observed for the binding to a/
that in an untreated control (Fig. 6, lane a). In addition,
b-tubulin. Firstly, a reduction in the binding of g-tubulin was
immunoprecipitation of tubulin at 48C also led to higher co-
observed after etoposide treatment (Fig. 5C, lane b) and g-
immunoprecipitation of CK1d (Fig. 6A, lane b), indicating a
irradiation in asynchronously growing cells (Fig. 5C, lane c).
strong association of CK1d to unpolymerized tubulin in vitro.
Secondly, etoposide treatment shortly after release from the
To confirm that increasing complex formation between
thymidine/aphidicolin-induced G1/S block led to a decrease of
CK1d and tubulin after disruption of microtubules by micro-
the binding of CK1d to g-tubulin in mitotic cells (Fig. 5C,
tubule-destabilising drugs also occurs in vivo, we analysed
lane e). However, similar to the situation with a/b-tubulin we
nocodazole and vinblastine-treated MEFs by laser scan
observed an increase in the co-precipitated amount of CK1d
microscopy (Fig. 6B). Staining of MEFs after 2 h treatment
(Fig. 5 C, lane f) with g-tubulin at mitosis, if cells had been g-
with nocodazole resulted in a total disruption of the micro-
irradiated in S phase. As a control we probed the same tubulin-
tubule network and led to a partial co-localisation of CK1d
immunoprecipitates for the tumor suppressor protein p53. In
with unpolymerized tubulin (Fig. 6B, c). However, we ob-
comparison to CK1d complex formation with tubulin we
served an even more pronounced co-staining of CK1d (Fig. 6B,
observed a uniform p53 signal under various physiological
b,e) and tubulin (Fig. 6B, a,d) after treatment of cells for 5 h
conditions, indicating an unspecific binding of p53 to tubulin
with vinblastine, which leads to the building of tubulin para-
(Fig. 5D, lanes a ± f).
crystals (Bensch and Malawista, 1968). The images of vinblas-
tine-treated cells show a clear co-localisation of CK1d with most
Microtubule-effecting drugs influence the
tubulin paracrystals in the merged laser scan image (Fig. 6B, f).
complex formation of CK1d and tubulin
In a modified assay we asked whether binding of CK1d to
CK1d phosphorylates tubulin isoforms, MAP4,
tubulin needs an assembled microtubule network. Therefore,
tau and stathmin in vitro
we considered the role of microtubule-effecting drugs in the
The association of CK1d with interphase microtubules and the
complex formation. Immunoprecipitation of tubulin from
spindle fibres of DNA-damaged cells indicates a functional
Balb/c 3T3 cellular extracts at 228C in the presence of the
role for CK1d in microtubule dynamics. Phosphorylation
microtubule-destabilizing drugs nocodazole (Fig. 6A, lane c)
events play a critical role in the regulation of the turnover
or vinblastine (Fig. 6A, lane d) led to a significant increase in
rate of microtubules. Especially microtubule-associated pro-
teins (MAPs) and stathmin, a small regulatory phosphoprotein,
influence the stability of microtubules (Gavet et al., 1998;
Moreno and Avila, 1998). Therefore, we analysed, if MAPs,
stathmin or even tubulins themselves are substrates for CK1d.
In vitro kinase assays were performed as described in
Material and methods. Purified bovine tubulin (ICN),
MAP4640±1125, tau and stathmin, respectively, were incubated
in the presence of [g-32P]ATP and the C-terminal truncated
CK1d or the GST-CK1d fusion protein FP449 (data not shown).
Proteins were separated by SDS-PAGE and visualized by
Coomassie staining (Fig. 7A ± D, a, b) and by autoradiography
(Fig. 7,A ± D, c,d). In control reactions no incorporation of
phosphate into a/b-tubulin was detected (Fig. 7A ± D, c). Strong
signals indicated that both, the C-terminal truncated CK1d
(Fig. 7A ± D, d) as well as the GST-CK1d fusion protein FP449
(data not shown), were able to phosphorylate a-tubulin, b-
tubulin, MAP4640±1125, tau and stathmin in vitro. To obtain further
information about the phosphorylation site(s), tryptic phospho-
peptides of a-tubulin, b-tubulin, MAP4640±1125, tau and stathmin
were analysed by two-dimensional tryptic phosphopeptide
analysis. The phosphorylated proteins were separated by SDS-
PAGE, blotted, digested with trypsin, oxidized and analysed in
Fig. 6. The effects of the microtubule-destabilising drugs nocodazole
two dimensions as described in Material and methods. In each
and vinblastine on the CK1d/tubulin complex formation and on the
case we observed several major and some minor phosphory-
subcellular distribution of CK1d. BalbC/3T3 cells were lysed and an a/
lated tryptic peptides, indicating that CK1d phosphorylates
b-tubulin-specific antibody was used to immunoprecipitate tubulin at
several amino acids in the proteins analysed (Fig. 7E).
228C (A, a, c, d) or 48C (A, b) in the presence (A, c, d) or absence (A, a,
b) of the microtubule-destabilising drugs. The immunoprecipitates
were separated by SDS-PAGE, blotted onto nitrocellulose and probed
for CK1d. a: control 228C, b: cold treatment 48C, c: nocodazole (5 mM,
228C ), d: vinblastine (2 mg/ml, 228C). MEFs were grown on coverslips
and treated with 5 mM nocodazole for 17 hours. After fixation the
We here provide evidence that CK1d interacts with different
localisation of CK1d and tubulin was analysed by immunofluorescence
cellular targets. CK1d co-localises with the TGN and interacts
(B, a ± c). In addition, cells were treated with 2 mg/ml vinblastine (B, d ±
f), respectively. The immunostaining for CK1d is shown in b and e, for
with microtubules in interphase cells. During mitosis CK1d is
a/b-tubulin in a and d and the superimposition of the CK1d and tubulin
evenly distributed throughout the cytoplasm, but upon DNA
staining in c and f.
damage the association of CK1d with the mitotic spindle is
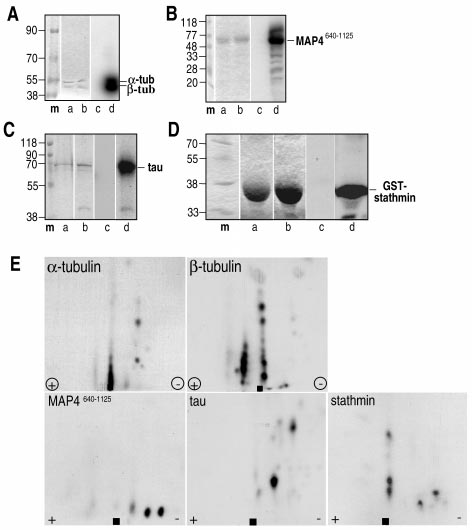
248 L. Behrend, G. Rutter et al.
Fig. 7. a- and b-tubulin, MAP4640±1125, tau and stathmin are phos-
PAGE, visualised by Coomassie staining (A ± D, lanes a, b) and by
phorylated by CK1d in vitro. Bovine tubulin, MAP4640±1125, tau and
autoradiography (A ± D, lanes c, d). m: molecular weight marker. For
GST-stathmin, respectively, were used as substrates for the in vitro
two-dimensional phosphopeptide analysis proteins phosphorylated by
phosphorylation by the C-terminal truncated CK1d (A ± D, b, d) in the
CK1d were separated by SDS-PAGE and blotted onto nitrocellulose.
presence of [g-32P]ATP. Control reactions were performed in the
Tryptic peptides were prepared and spotted onto thin layer plates at the
absence of the kinase (A ± D, a, c). Proteins were separated by SDS-
origin (marked with a square).
specifically activated, indicating that CK1d might play a role in
obvious anterograde redistribution, whereas after long-term
regulatory processes during mitosis.
treatment the CK1d-stained structures collapsed to aggregates
Our analysis of the localisation of CK1d in interphase cells
in the MTOC region. The observed alterations during BFA
by laser scan microscopy revealed that CK1d antibodies
exposure are similar to the changes described for the TGN
stained predominantly perinuclear membrane structures
during BFA treatment (Ladinsky and Howell, 1992; Lippin-
which were sensitive to detergent treatment. During short-
cott-Schwartz et al., 1991; Strous et al., 1993). Indeed, co-
time exposure to BFA these reticular membranes showed an
staining of CK1d with TGN38, an integral membrane protein
Interaction of CK1d with post-Golgi strucures, microtubules and the spindle apparatus 249
of the TGN, in various cell lines of rodent origin clearly showed
believed to be controlled by phosphorylation. In this study
that CK1d associates with the TGN. In addition, we found that
we present data that CK1d is able to phosphorylate several
CK1d co-localises with g-adaptin in the perinuclear region. g-
amino acids of a- and b-tubulin. Phosphorylation of tubulins is
Adaptin as component of the adapter complex AP1 resides to
thought to be one of the principal mechanisms adjusting the
clathrin-coated regions of the TGN that drive vesicle budding
stability of microtubules (Aletta, 1996; MacRae, 1997). The
(Sosa, 1996; Wong and Brodsky, 1992). Vesicle generation in
CK1d-mediated phosphorylation of a/b-tubulin might influ-
the trans Golgi network is an important site for the sorting of
ence the turnover rate of microtubules and thereby regulating
proteins addressed to the plasma membrane, secretory vesi-
the rebuilding of microtubules during mitosis.
cles, or lysosomes (Keller and Simons, 1997). The association
In addition to a/b-tubulin, CK1d phosphorylates MAPs like
of CK1d with the TGN and the partial co-staining of CK1d
MAP4 and tau (Kuret et al., 1997; Singh et al., 1995, our own
with g-adaptin suggests that CK1d participates in regulating
data) as well as stathmin. Both, expression level and phos-
the budding of clathrin-coated vesicles and consequently
phorylation status of MAPs are known to be involved in the
influences sorting functions of the TGN. Kinase and phospha-
regulation of microtubule stability (Drewes et al., 1998;
tase activities have been shown to regulate the interaction of
Moreno and Avila, 1998). Therefore, one might predict that
adapter proteins with clathrin and thereby modulating the
CK1d is involved in the control of M phase-specific rebuilding
dynamics of vesicle formation (Chen et al., 1999; Sabatini
of the microtubule network. Further studies will be needed to
et al., 1996; Wilde and Brodsky, 1996). An involvement of CK1
clarify the character of the implication of CK1d in these
family members in TGN-specific functions has been described.
For example, CK1a is associated with the TGN and partici-
Other isoforms of the CK1 family have also been shown to
pates in the regulation of vesicular trafficking (Gross and
be involved in chromosome segregation during mitosis and
Anderson, 1998; Gross et al., 1997). Recently, it has been
meiosis. For example, the a isoform is located at the spindle
shown that the yeast homologue hrr25p of CK1d is involved in
and the centrosomes in both mouse fibroblasts and oocytes
the regulation of vesicle budding from the ER in yeast
(Brockman et al., 1992; Gross et al., 1997). So far, in all of these
(Murakami et al., 1999). However, further experiments are
studies the association with the cell division machinery has
necessary to prove an involvement of CK1d in the regulation
been described as being constitutive. In contrast, we here
of TGN-specific processes.
demonstrate that CK1d only associates to a significant amount
Besides the perinuclear region, antibodies against CK1d
with the spindle and the centrosomes when induced by
stained granular particles in the cytoplasm, which seem to be
genotoxic stress, thereby underlining the involvement of
aligned in linear arrays. Extraction of soluble proteins of cells
CK1d in stress signalling pathways. The fact that CK1d
prior to fixation led to immunofluorescence images that clearly
especially phosphorylates p53, a major stress integrator
localise these granular particles to microtubules. These find-
protein of the cell, at G2/M (Meek, 1998) (our own unpub-
ings, confirmed by our immunoelectron microscopy data, point
lished data) suggests that such a phosphorylation event could
to a close association of CK1d with tubulin. Indeed, we were
form the basis for a signal to p53 indicating the status of mitotic
able to co-immunoprecipitate CK1d with antibodies specific
progression. Further studies are in process to characterise this
for different isoforms of tubulin even in the presence of the
microtuble-destabilizing drugs nocodazole and vinblastine.
In summary, our analyses of the cell cycle-specific localisa-
Therefore, we assume that CK1d is able to interact with
tion of CK1d provide new clues to the function of CK1d. The
tubulins, either directly or mediated by an unknown linker.
mechanism controlling the spindle association of CK1d in
The association of CK1d with microtubules and in addition
response to cellular stress and the consequences for cell cycle
with membrane structures of the TGN suggests a participation
progression remain to be elucidated.
of CK1d in microtubule-membrane interactions. This could
Acknowledgements. We are grateful to our colleagues who have kindly
indicate that CK1d plays a role in microtubule-dependent
provided us with important reagents. These include Merl Hoekstra and
transport processes. This view is also supported by the fact that
Toni DeMaggio (ICOS Corporation, Washington, USA) (monoclonal
CK1d possesses a kinesin homology domain (Roof et al., 1992;
anti-CK1d antibody 128A), George Banting (anti-TGN38), Annette
Xu et al., 1995), shown to be responsible for the interaction of
Hille-Rehfeld (anti-cathepsin A), Eva-Maria Mandelkow (MAP4640±1125,
the motor protein kinesin with tubulin. Furthermore, it has
tau), Irene Dornreiter and Silke Dehde (GST-stathmin) and David
been shown that CK1a participates in vesicle transport
Meek (GST-fusion proteins FP449 and FP455). We thank Tilo
processes (Gross and Anderson, 1998; Gross et al., 1995).
Patschinsky and Christine Schulze-Garg for helpful discussion and
The interaction of CK1d with tubulin is not restricted to
reading of the manuscript. This work was supported by a grant from the
interphase but is also found during mitosis. While untreated
Deutsche Krebshilfe, Dr. Mildred Scheel Stiftung, to Uwe Knippschild
(10 ± 1285-Kn I). The Heinrich-Pette-Institut is financially supported by
mitotic cells showed no spindle association of CK1d in the
Freie und Hansestadt Hamburg and Bundesministerium für Gesund-
immunofluorescence microscopy, treatment with either etopo-
heit. This work is part of the PhD thesis of Lars Behrend, Fachbereich
side or g-irradiation at the onset of S-phase induces a strong
Biologie at the University of Hamburg.
particular alignment of CK1d with the mitotic spindle and
centrosomes (Fig. 4). The finding that CK1d is recruited to the
spindle in response to DNA damage is also supported by our
binding affinity data. The complex formation of CK1d with a/
b-tubulin and g-tubulin was dramatically enhanced in extracts
from mitotic cells after exposure to genotoxic stress during S
Aaronson, S. A., Todaro, G. J. (1968): Development of 3T3-like lines
from Balb/C mouse embryo cultures: Transformation susceptibility
phase. The increased complex formation of the stress-activated
to SV40. J. Cell. Physiol. 72, 141 ± 148.
CK1d with spindle components provides evidence, that CK1d
Aletta, J. M. (1996): Phosphorylation of type III beta-tubulin PC12 cell
is involved in rearrangement of the microtubule network at the
neurites during NGF-induced process outgrowth. J. Neurobiol. 31,
onset of mitosis. Changes of microtubule dynamics are
250 L. Behrend, G. Rutter et al.
Bensch, K. G., Malawista, S. E. (1968): Microtubule crystals: a new
of casein kinase 1 and enhances the level of casein kinase 1 delta in
biophysical phenomenon induced by Vinca alkaloids. Nature 218,
response to topoisomerase-directed drugs. Oncogene 15, 1727 ±
1176 ± 1177.
Brockman, J. L., Gross, S. D., Sussman, M. R., Anderson, R. A. (1992):
Kuret, J., Johnson, G. S., Cha, D., Christenson, E. R., DeMaggio, A. J.,
Cell cycle-dependent localization of casein kinase I to mitotic
Hoekstra, M. F. (1997): Casein kinase 1 is tightly associated with
spindles. Proc. Natl. Acad. Sci. USA 89, 9454 ± 9458.
paired-helical filaments isolated from Alzheimers disease brain. J.
Cegielska, A., Gietzen, K. F., Rivers, A., Virshup, D. M. (1998):
Neurochem. 69, 2506 ± 2515.
Autoinhibition of casein kinase I epsilon (CKI epsilon) is relieved
Ladinsky, M. S., Howell, K. E. (1992): The trans-Golgi network can be
by protein phosphatases and limited proteolysis. J. Biol. Chem. 273,
dissected structurally and functionally from the cisternae of the
1357 ± 1364.
Golgi complex by brefeldin A. Eur. J. Cell Biol. 59, 92 ± 105.
Chen, H., Slepnev, V. I., Di Fiore, P. P., De Camilli, P. (1999): The
Lingle, W. L., Lutz, W. H., Ingle, J. N., Maihle, N. J., Salisbury, J. L.
interaction of epsin and Eps15 with the clathrin adaptor AP-2 is
(1998): Centrosome hypertrophy in human breast tumors: implica-
inhibited by mitotic phosphorylation and enhanced by stimulation-
tions for genomic stability and cell polarity. Proc. Natl. Acad. Sci.
dependent dephosphorylation in nerve terminals. J. Biol. Chem. 274,
USA 95, 2950 ± 2955.
3257 ± 3260.
Lippincott-Schwartz, J., Yuan, L., Tipper, C., Amherdt, M., Orci, L.,
Christenson, E., DeMaggio, A. J., Hockstra, M. F. (1997): The role of
Klausner, R. D. (1991): Brefeldin As effects on endosomes,
workhorse protein kinases in coordinating DNA metabolism and
lysosomes, and the TGN suggest a general mechanism for regulating
cell growth. Rec. Res. Cancer Res. 143, 263 ± 274.
organelle structure and membrane traffic. Cell 67, 601 ± 616.
Dhillon, N., Hoekstra, M. F. (1994): Characterization of two protein
Longenecker, K. L., Roach, P. J., Hurley, T. D. (1998): Crystallographic
kinases from Schizosaccharomyces pombe involved in the regulation
studies of casein kinase I delta toward a structural understanding of
of DNA repair. EMBO J. 13, 2777 ± 2788.
auto-inhibition. Acta Crystallogr. D Biol. Crystallogr. 54, 473 ± 475.
Drewes, G., Ebneth, A., Mandelkow, E. M. (1998): MAPs, MARKs and
MacRae, T. H. (1997): Tubulin post-translational modifications ±
microtubule dynamics. Trends Biochem. Sci. 23, 307 ± 311.
enzymes and their mechanisms of action. Eur. J. Biochem. 244,
Drubin, D. G., Nelson, W. J. (1996): Origins of cell polarity. Cell 84,
Meek, D. W. (1998): New developments in the multi-site phosphoryla-
Fish, K. J., Cegielska, A., Getman, M. E., Landes, G. M., Virshup, D. M.
tion and integration of stress signalling at p53. Int. J. Radiat. Biol. 74,
(1995): Isolation and characterization of human casein kinase I
epsilon (CKI), a novel member of the CKI gene family. J. Biol.
Moreno, F. J., Avila, J. (1998): Phosphorylation of stathmin modulates
Chem. 270, 14875 ± 14883.
its function as a microtubule depolymerizing factor. Mol. Cell.
Freeman, A. E., Igel, H. J., Price, P. J. (1975): Carcinogenesis in vitro. I.
Biochem. 183, 201 ± 209.
In vitro transformation of rat embryo cells: Correlations with the
Moritz, M., Braunfeld, M. B., Sedat, J. W., Alberts, B., Agard, D. A.
known tumorigenic activity of chemicals in rodents. In Vitro 2, 107 ±
(1995): Microtubule nucleation by gamma-tubulin-containing rings
in the centrosome. Nature 378, 638 ± 640.
Gavet, O., Ozon, S., Manceau, V., Lawler, S., Curmi, P., Sobel, A.
Murakami, A., Kimura, K., Nakano, A. (1999): The inactive form of a
(1998): The stathmin phosphoprotein family: intracellular localiza-
yeast casein kinase I suppresses the secretory defect of the sec12
tion and effects on the microtubule network. J. Cell. Sci. 111, 3333 ±
mutant. Implication of negative regulation by the Hrr25 kinase in
the vesicle budding from the endoplasmic reticulum. J. Biol. Chem.
Graves, P. R., Haas, D. W., Hagedorn, C. H., DePaoli-Roach, A. A.,
274, 3804 ± 3810.
Roach, P. J. (1993): Molecular cloning, expression, and character-
Panek, H. R., Stepp, J. D., Engle, H. M., Marks, K. M., Tan, P. K.,
ization of a 49-kilodalton casein kinase I isoform from rat testis. J.
Lemmon, S. K., Robinson, L. C. (1997): Suppressors of YCK-
Biol. Chem. 268, 6394 ± 6401.
encoded yeast casein kinase 1 deficiency define the four subunits
Graves, P. R., Roach, P. J. (1995): Role of COOH-terminal phosphor-
of a novel clathrin AP-like complex. EMBO J. 16, 4194 ± 4204.
ylation in the regulation of casein kinase I delta. J. Biol. Chem. 270,
Peters, J. M., McKay, R. M., McKay, J. P., Graff, J. M. (1999): Casein
21689 ± 21694.
kinase I transduces Wnt signals. Nature 401, 345 ± 350.
Gross, S. D., Anderson, R. A. (1998): Casein kinase I: spatial organiza-
Roach, P. J. (1990): Control of glycogen synthase by hierarchal protein
tion and positioning of a multifunctional protein kinase family [In
phosphorylation. FASEB J. 4, 2961 ± 2968.
Process Citation]. Cell Signal 10, 699 ± 711.
Robinson, L. C., Menold, M. M., Garrett, S., Culbertson, M. R. (1993):
Gross, S. D., Hoffman, D. P., Fisette, P. L., Baas, P., Anderson, R. A.
Casein kinase I-like protein kinases encoded by YCK1 and YCK2
(1995): A phosphatidylinositol 4,5-bisphosphate-sensitive casein
are required for yeast morphogenesis. Mol. Cell Biol. 13, 2870 ± 2881.
kinase I alpha associates with synaptic vesicles and phosphorylates
Roof, D. M., Meluh, P. B., Rose, M. D. (1992): Kinesin-related proteins
a subset of vesicle proteins. J. Cell. Biol. 130, 711 ± 724.
required for assembly of the mitotic spindle. J. Cell Biol. 118, 95 ± 108.
Gross, S. D., Simerly, C., Schatten, G., Anderson, R. A. (1997): A casein
Rowles, J., Slaughter, C., Moomaw, C., Hsu, J., Cobb, M. H. (1991):
kinase I isoform is required for proper cell cycle progression in the
Purification of casein kinase I and isolation of cDNAs encoding
fertilized mouse oocyte. J. Cell Sci. 110, 3083 ± 3090.
multiple casein kinase I-like enzymes. Proc. Natl. Acad. Sci. USA 88,
Hoekstra, M. F., Dhillon, N., Carmel, G., DeMaggio, A. J., Lindberg,
9548 ± 9552.
R. A., Hunter, T., Kuret, J. (1994): Budding and fission yeast casein
Sabatini, D. D., Adesnik, M., Ivanov, I. E., Simon, J. P. (1996):
kinase I isoforms have dual-specificity protein kinase activity. Mol.
Mechanism of formation of post Golgi vesicles from TGN mem-
Biol. Cell. 5, 877 ± 886.
branes: Arf-dependent coat assembly and PKC-regulated vesicle
Keller, P., Simons, K. (1997): Post-Golgi biosynthetic trafficking. J. Cell
scission. Biocell 20, 287 ± 300.
Sci. 110, 3001 ± 3009.
Sakanaka, C., Leong, P., Xu, L., Harrison, S. D., Williams, L. T. (1999):
Knippschild, U., Kolzau, T., Deppert, W. (1995): Cell-specific transcrip-
Casein kinase epsilon in the wnt pathway: regulation of beta-catenin
tional activation of the mdm2-gene by ectopically expressed wild-
function [In Process Citation]. Proc. Natl. Acad. Sci. USA 96,
type form of a temperature-sensitive mutant p53. Oncogene 11,
12548 ± 12552.
Schwartz, D., Almog, N., Peled, A., Goldfinger, N., Rotter, V. (1997):
Knippschild, U., Milne, D., Campbell, L., Meek, D. (1996): p53 N-
Role of wild type p53 in the G2 phase: regulation of the gamma-
terminus-targeted protein kinase activity is stimulated in response to
irradiation-induced delay and DNA repair. Oncogene 15, 2597 ±
wild type p53 and DNA damage. Oncogene 13, 1387 ± 1393.
Knippschild, U., Milne, D. M., Campbell, L. E., DeMaggio, A. J.,
Schwartz, D., Rotter, V. (1998): p53-dependent cell cycle control:
Christenson, E., Hoekstra, M. F., Meek, D. W. (1997): p53 is
response to genotoxic stress [In Process Citation]. Semin. Cancer
phosphorylated in vitro and in vivo by the delta and epsilon isoforms
Biol. 8, 325 ± 336.
Interaction of CK1d with post-Golgi strucures, microtubules and the spindle apparatus 251
Singh, T. J., Grundke-Iqbal, I., Iqbal, K. (1995): Phosphorylation of tau
Wong, D. H., Brodsky, F. M. (1992): 100-kD proteins of Golgi- and
protein by casein kinase-1 converts it to an abnormal Alzheimer-like
trans-Golgi network-associated coated vesicles have related but
state. J. Neurochem. 64, 1420 ± 1423.
distinct membrane binding properties. J. Cell Biol. 117, 1171 ± 1179.
Sosa, M. A. (1996): The adaptor complexes: a bridge between the
Xu, R. M., Carmel, G., Sweet, R. M., Kuret, J., Cheng, X. (1995):
transmembrane proteins and clathrin lattices. Biocell 20, 301 ± 305.
Crystal structure of casein kinase-1, a phosphate-directed protein
Strous, G. J., van Kerkhof, P., van Meer, G., Rijnboutt, S., Stoorvogel,
kinase. EMBO J. 14, 1015 ± 1023.
W. (1993): Differential effects of brefeldin A on transport of
Zhai, L., Graves, P. R., Robinson, L. C., Italiano, M., Culbertson, M. R.,
secretory and lysosomal proteins. J. Biol. Chem. 268, 2341 ± 2347.
Rowles, J., Cobb, M. H., DePaoli-Roach, A. A., Roach, P. J. (1995):
Tuazon, P. T., Traugh, J. A. (1991): Casein kinase I and II ± multi-
Casein kinase I gamma subfamily. Molecular cloning, expression,
potential serine protein kinases: structure, function, and regulation.
and characterization of three mammalian isoforms and complemen-
Adv. Second Messenger Phosphoprotein Res. 23, 123 ± 164.
tation of defects in the Saccharomyces cerevisiae YCK genes. J. Biol.
Vaidya, A. B., Lasfargues, E. Y., Sheffield, J. B., Coutinho, W. G. (1978):
Chem. 270, 12717 ± 12724.
Murine mammary tumor virus (MuMTV) infection of an epithelial
Zhai, Y., Kronebusch, P. J., Simon, P. M., Borisy, G. G. (1996): Micro-
cell line established from C57BL/6 mouse mammary glands.
tubule dynamics at the G2/M transition: abrupt breakdown of
Virology 90, 12 ± 22.
cytoplasmic microtubules at nuclear envelope breakdown and
Wilde, A., Brodsky, F. M. (1996): In vivo phosphorylation of adaptors
implications for spindle morphogenesis. J. Cell Biol. 135, 201 ± 214.
regulates their interaction with clathrin. J. Cell Biol. 135, 635 ± 645.
Source: http://www.ck1-lab.de/publi/fullBehrend2000a.pdf
Detecting Bacillus Spores by Raman and Surface-Enhanced Raman Spectroscopy (SERS) Raman spectroscopy has been employed to detect Bacillus cereusspores, an anthrax surrogate, collected from a letter as it passed Intensity (arbitrary units) through a mail sorting system. Raman spectroscopy also has the capability to identify many common substances used as hoaxes. A
Memoria de Master en Didáctica del español L2/LE Universidad de la Rioja Edición 2006 -2008 LOS ACTOS DE HABLA EN LA ENSEÑANZA DE ESPAÑOL COMO LENGUA EXTRANJERA: EL CASO DE LA SUGERENCIA Asesora de la memoria: Cristina Vela Delfa Autora: Cristina Mosquera Blanco